The cultivation of lulo in Colombia is of great importance, and the international potential of this fruit is promising. The increase in the harvested area went from 7,039 to 8,655 ha between 2010 and 2016. In production, it rose from 59,091 to 78,610 t in the same period, demonstrating the interest of producers and the economic advantages that this fruit offers (Agronet, 2018). In addition, the recent free trade agreements (FTAs) signed with several countries around the world opened new markets for Colombian agriculture, which requires strong technological boosts in crops to make Colombia highly competitive, as seen in the case of the lulo.
Lulo producers in Colombia face the susceptibility of cultivars to pests and diseases, farm heterogeneity, and reduction of vegetative and reproductive development. Agronomic problems that limit productivity and, in some cases, cause total crop loss (Lobo, 2006; Cruz et al., 2007; Muñoz, 2013). Despite the losses caused by these problems, the cultivation area and production increased by 7.58% and 3.34%, respectively, in Nariño (Colombia) between 2010 and 2016 (Agronet, 2018). By searching suitable culturable areas, the low technological level of lulo farming systems has caused deforestation, making it necessary to rethink current cultivation techniques in order to avoid environmental problems (Muñoz, 2013).
These drawbacks mean that breeding programs aimed at increasing productivity are needed. It pursues lulo varieties tolerant to the major diseases and greater efficiency in transforming light into chemical energy, along with better distribution of carbohydrates (Angulo, 2006).
In Colombia, minimal efforts in lulo breeding have been geared toward obtaining cultivars resistant or tolerant to pathogens and pest, such as Fusarium sp., Phytophthora infestans, Neolucinodes elegantalis, and Meloidogyne sp. It has been necessary to resort to related species and obtain hybrids with resistance, but with lower quality, since all lulo varieties show susceptibility (Betancourth et al., 2005; Muñoz, 2013). Despite its low domestication, the lulo has stability in specific niches and restrict general adaptability (Lobo, 2006).
Genetic variability is essential in plant breeding programs, it can be a definitive solution in crop breeding, especially in crops with limited variability (Subramanian, 2011; Rimieri, 2017). The induction of mutations is a widely used method to produce genetic variability, but it is necessary to evaluate the effectiveness and efficiency of each technique for the frequency of desirable mutants before considering one a potent tool for the improvement of plants (Prina et al., 2010).
Artificial mutagenic agents can be physical or chemical. Treatments with ionizing radiation produce high degrees of chromosomal damage with breaks in the DNA chain and physiological effects that cause cell death and sterility in first-generation plants. Currently, the most commonly used radiation for plant breeding purposes includes x-rays and gamma rays (Sonone et al., 2010; Prina et al., 2010; Velmurugan et al., 2010; Silvera, 2017). However, the mutation rate can also be increased by in vitro cultures (somaclonal variation). It is possible to select plants by obtaining agronomically useful mutants and combine mutation techniques with in vitro propagation (Patade et al., 2008).
The improvement of the tolerance to water stress conditions of many crops has been achieved with in vitro selection of mutants obtained with radiation or chemical methods. These procedures have been tested on multiple crops, such as potatoes (Velmurugan et al., 2010), peanuts (Sonone et al., 2010), soybeans (Satpute and Fultambkar, 2012; Kavithamani et al., 2010; Ortiz et al., 2008), rice (Baloch et al., 2004), sorghum (Jayaramachandran et al., 2010), cherries (Roman et al., 2009), sunflower (Kumar and Ratnam, 2010), sugar cane (Singh, 1993; Desai, 2006), among others. The improvement of crops with genetic mutations has also been successful in obtaining new colors and shapes in ornamental plants, and many mutants are induced by gamma radiation and other mutagens that are currently marketed (Datta et al., 2005; Matsumura et al., 2010; Sahariya et al., 2017)
Mutagenic induction with chemicals and radiation has been successfully used to obtain desirable agronomic characteristics. The 89% of mutant varieties have been obtained with irradiation methods, from which 64% were obtained using gamma radiation (Patade, 2008).
According to Predieri and Zimmerman (2001) and Mostafa et al. (2015), the effectiveness and efficiency of irradiation depend on the dose used, the genotype, and the type of irradiated explant. Therefore, the present study aimed to carry out an in vitro evaluation of the effect of Co60 gamma ray irradiation on the somaclonal variation of lulo (Solanum quitoense Lam) with and without thorns.
MATERIALS AND METHODS
This study was carried out in the Tissue Laboratory of the Biology program of the Faculty of Sciences at the Universidad Nacional de Colombia (Medellín Headquarters); the gamma radiation was carried out in the nuclear reactor of the Instituto Colombiano de Geología y Minería (INGEOMINAS), using a Co60 source.
The plants of lulo with and without thorns were obtained with three subcultures in an MS medium and calluses produced with foliar segments of lulo with thorns in an MS medium supplemented with ANA (6.0 mg L-1) and sucrose at 9%, which were incubated in darkness for 45 days, and then were irradiated.
The plants and calluses were cultivated in Petri dishes (Ø=9 cm) in an MS medium before being irradiated in a pilot irradiation plant, with an activity of 10000 curies and a cobalt60 source; the irradiation doses were 0 Gy (Control), 15 Gy, 30 Gy, 45 Gy, and 60 Gy. Immediately after the treatment, the plants and calluses were transplanted to containers with a fresh MS culture medium. A completely randomized design, with a bifactorial arrangement, was used to analyze the irradiated plants' prolificacy and survival. The factors corresponded to the two varieties of lulo (Factor A) and the five doses of irradiation (Factor B). Each irradiated treatment (plants and callus) consisted of eight repetitions (Petri dishes), with four explants each.
To analyze the effect of the irradiation on the genetic fidelity, foliar samples were taken from the live explants after six months for DNA extraction following the methodology of Dellaporta et al. (1983) at a concentration of 10 ng μL-1, based on dilutions of phage lambda DNA (Sigma, St. Louis, MO, USA).
In the PCR, eight primers were tested, and seven were analyzed, considering that the CGA primer showed no amplification. The primers used in the RAM technique to determine the effect of the gamma irradiation on the lulo plants and calluses are presented in Table 1.
Table 1 Primers and sequences used in the RAM technique to determine the genetic stability of the lulo seedlings and calluses irradiated with gamma rays.
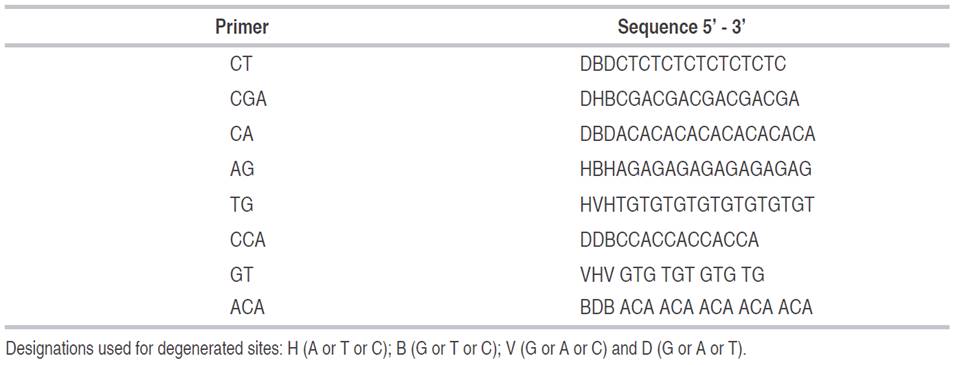
The hybridization and amplification conditions were established according to the selected primer (Table 2). The products were separated and visualized in 7% polyacrylamide gels (37:1 acrylamide-bisacrylamide) ran at 160 V for 1 h and 10 min, and stained with ethidium bromide and silver salts as described by Sambrook et al. (1989).
The observation of the gels provided a binary matrix for presence (1) and absence (0). Based on this matrix, descriptive analyses were carried out, and the genetic distances and similarities were established. The unbiased minimum distance criterion (Nei, 1978) was used for the classification analysis following the UPGMA method. These analyses were carried out using TFPGA (Tools for Population Genetic Analysis), as described by Miller (1997).
RESULTS AND DISCUSSION
Survival
The analysis of variance for the percentage of live explants two months after the irradiation showed highly significant differences between the lulo varieties, between the irradiation doses and for the variety×dose interaction (Table 3). At the variety level, the lulo with thorns presented greater survival at the higher radiation doses, requiring an approximate dose of 28 Gy to cause 50% mortality. The lulo variety without thorns proved to be more susceptible to irradiation, with the same level of mortality at a radiation dose of 19 Gy (Figure 1). In general, the plants submitted to high doses presented foliar chlorosis, necrosis of the basal regions of the petioles, leaf fall, growth arrest, and death.
Table 3 Analysis of variance for the survival and regeneration capacity variables of lulo seedlings subjected to different doses of radiation with gamma rays.
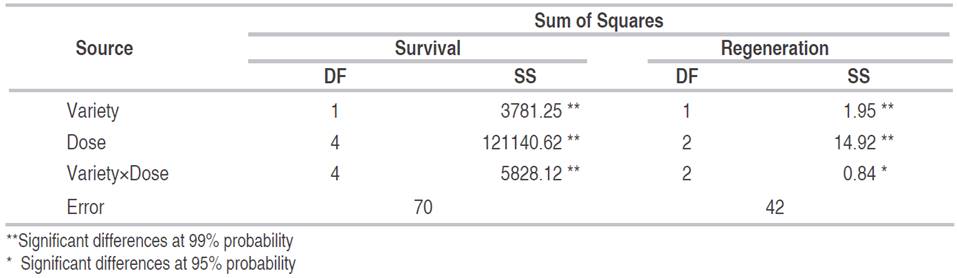
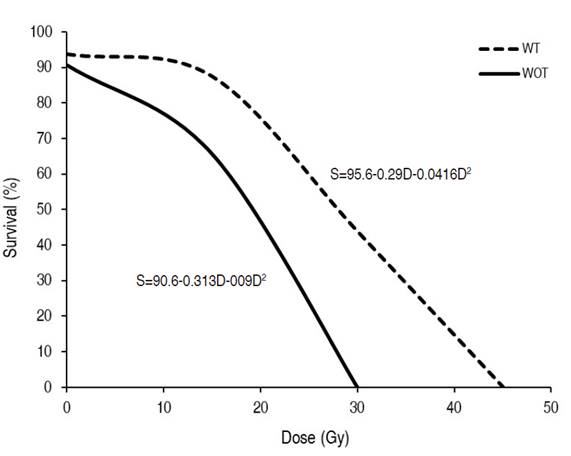
Figure 1 Survival percentage of lulo seedlings (Solanum quitoense Lam.) with thorns (WT) and without thorns (WOT) subjected to different doses of gamma radiation with Co60.
The reaction among the lulo varieties has been observed in other species, such as soybeans (Satpute and Fultambkar, 2012), mung bean (Singh, 2007), rice (Baloch, et al., 2004), beans (Rocha et al., 2010) and pears (Predieri and Zimmerman, 2001). These differences can be attributed to the differential sensitivity of plants to radiation, because their adaptation to light (WT) and understory (WOT) conditions, may affect physiological properties at the cellular and nuclear level (Baloch et al., 2004; Rocha et al., 2010); an examination of the leaf histology indicates that the two varieties present very similar characteristics (Medina et al., 2008)
Multiplication capacity
The regeneration capacity of the plants from the initial explants after irradiation presented statistical differences between the varieties, irradiation dose and in the varietyxdose interaction (Table 3). At the variety level, the lulo with thorns had a greater multiplication capacity (1.52 seedlings per explant) than the thornless variety (1.12 seedlings per explant), confirming that the latter is more radiosensitive since, at the control level (0 Gy), they had a similar behavior (2.33 seedlings per explant and 2.37 seedlings per explant, respectively). The ability of the initial explants to regenerate plants in the first 30 days after irradiation was correlated with the intensity; as the irradiation dose increased, the prolificacy of the explants was markedly reduced (Figure 2). The radiation-induced necrosis caused this result at the meristematic level, which caused general growth arrest that prevented or reduced the formation of appropriate phytomers for multiplication.
Genetic Variability
The band count for the seven primers showed 115 bands for the irradiated seedlings and 114 for the irradiated calluses; the number of bands was between 12 for the TG primer and 21 for the ACA primer (Figure 3).
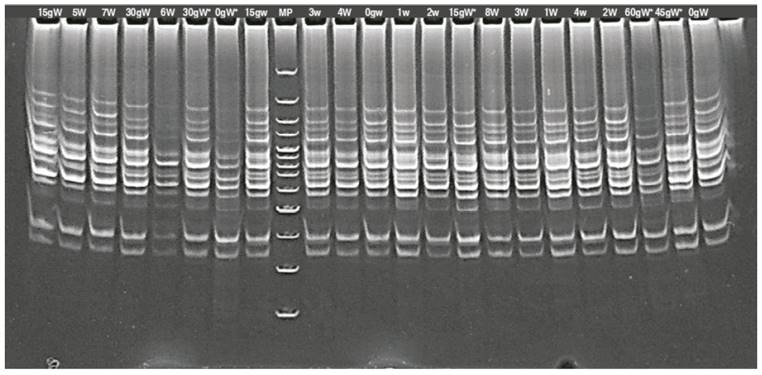
Figure 3 Banding patterns generated with RAM primer in lulo (Solanum quitoense Lam.) calluses with thorns (*) and in lulo seedlings with (W) and without (w) thorns, irradiated with gamma rays. g: indicate the irradiation doses; MP: marker.
The lulo calluses irradiated with different doses of gamma rays showed average unbiased heterozygosity of 0.22 and polymorphism of 50%. The analysis of the genetic distances provided values between 0.40 (between 0 Gy and 30 Gy) and 0.17 (between 0 Gy and 60 Gy). Likewise, the control treatment (without irradiation) presented similar values of 0.66 and 0.84 when compared with the irradiation doses of 30 Gy and 60 Gy, respectively (Table 4). The observed variability confirmed the effectiveness of gamma radiation in the induction of genetic variants at the level of the treated lulo explants (callus) and the sensitivity of the species to gamma radiation. This sensitivity depends on factors such as the physiological properties of the cells and nuclei, DNA content, chromosome size and ploidy level (Baloch et al., 2004, Subramanian et al., 2011).
Table 4 Matrix of genetic distance (above the diagonal) of Nei (1978) and similarity (below the diagonal) obtained in lulo with thorns calluses irradiated with Co60 gamma rays.
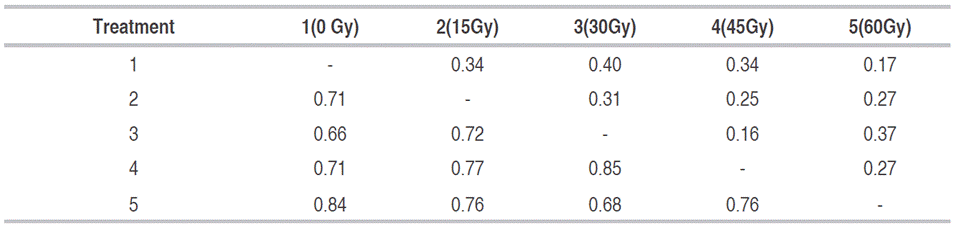
The cluster analysis using the UPGMA criterion with Nei's distances (1972) formed four nodes. Node 1, with a distance of 0.16, contained the doses 30 Gy and 45 Gy, which, along with the 15 Gy dose, formed the second node, while the control dose (0 Gy) and the maximum dose of 60 Gy formed a third group with a distance of 0.17. All treatments were included in the fourth node (Figure 4).
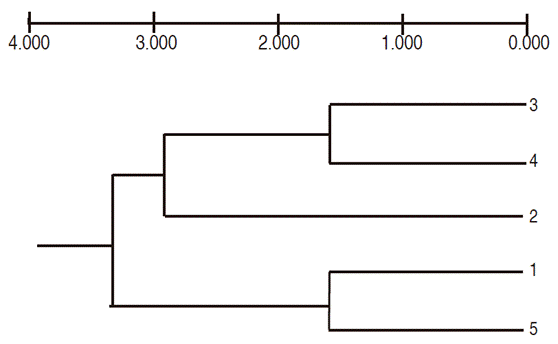
Figure 4 Dendogram of the genetic variability of calluses induced with lulo cotyledonary leaves and irradiated with Co60 gamma ray doses (Based on Nei's distances, combined data of seven RAM primers and UPGMA classification criteria; 1 = 0 Gy, 2 = 15 Gy, 3 = 30 Gy, 4 = 45 Gy, 5 = 60 Gy).
The analysis of the irradiation effect on lulo seedlings with and without thorns revealed unbiased heterozygosity of 0.13 and polymorphism of 31.03%; heterozygosity estimates the probability that two randomly drawn alleles from a set of genes in a population are different. The greatest genetic distance (0.22) was observed between the lulo with and without thorns controls, with a similarity of 0.80. The distance between the lulo with thorns control and the doses 15 Gy and 30 Gy was 0.15 and 0.18, with a similarity of 85% and 83%, respectively. There was a genetic distance of 0.15 and a similarity of 85% between the lulo without thorns control and the dose 15 Gy. The heterogeneity of responses to radiation between species and between genotypes of the same species was reported by Arena et al. (2017), attributed mainly to differences between DNA contents (Subramanian et al., 2011); however, the IAEA (1977) stated that radiosensitivity differences between genotypes of the same species are much lower than between other species.
The cluster analysis formed four groups. The treatments with 15 Gy on lulo without thorns and with 30 Gy on lulo with thorns formed group one, located at a distance of 0.13, which, along with the control treatment without thorns, formed group two at a distance of 0.17. Group three contained the treatments 0 Gy and 15 Gy with thorns at a distance of 0.14, and group four included all treatments at a distance of 0.18 (Figure 5).
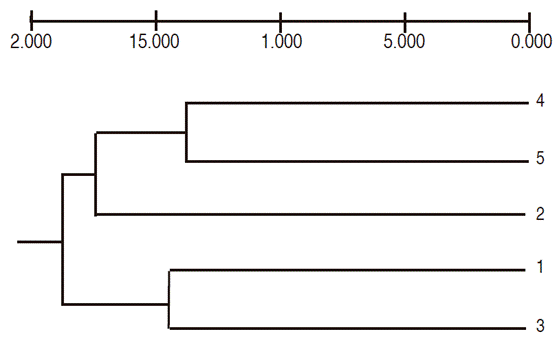
Figure 5 Dendogram of the genetic variability of lulo seedlings with (CE) and without thorns (SE) irradiated with different Co60 gamma ray doses (Based on Nei's distances, combined data of seven RAM primers and UPGMA classification criteria; 1 = 0Gy CE, 2 = 0Gy SE, 3 = 10Gy CE, 4 = 15Gy SE, 5 = 30Gy SE).
CONCLUSIONS
The Gamma ray irradiation of calluses induced from cotyledonary leaves of lulo and seedlings regenerated from lulo with and without thorns, generates genetic variability. The survival of the explants at the different doses of gamma radiation showed that the calluses were less radiosensitive (28 Gy, 50% mortality) and the lulo seedlings without thorns, were the most sensitive to radiation (19 Gy, 50% mortality). The ability of the irradiated explants (seedlings) to regenerate plants was inversely proportional to the dose of gamma rays received; the effects of the radiation were genotype-dependent.