Arboviruses are viruses transmitted by arthropods that have received high public health attention worldwide (Weaver and Reisen, 2010). The most important diseases caused by arboviruses that infect humans are dengue, zika, yellow fever, and chikungunya (Shepardet al., 2014). These diseases are vector-transmitted by the insectAedes aegypti.However,Aedes albopictusis also a vector of the dengue virus (Kraemeret al., 2015).
These diseases caused by viruses are one of the main global health concern that has increased dramatically due to their rapid geographical spread, high disease burden, and distribution (Leta et al., 2018). The spread of the insect vector has reached areas beyond the tropics in recent years, causing a significant problem not only in undeveloped countries (Kraemer et al., 2015; Chadee and Martinez, 2016).
Artificial reservoirs such as old discarded tires, plant pots, uncovered water tanks, and untreated pools are the main oviposition sites forA. aegypti, where the female mosquitoes are attracted to deposit their eggs (Muktaret al., 2016). TheAedeslarvae can be distinguished from other genera with the naked eye due to their short siphon, used to breathe oxygen that is kept above the water surface while the rest of the body remains vertically immersed (Muktaret al., 2016). Males develop faster than females, and if temperature is cold,A. aegyptican stay in the larval stage for months, as long as the water supply is maintained (Muktaret al., 2016).
Several countries have developed plans to containA. aegypti, with the aim of reducing the infestation rates and the impacts caused by the viruses transmitted (Nashet al., 2017). Investing in measures that intend to control the transmitting vector and reduce their proliferation is as fundamental as the development of vaccines and diagnostic methods (Zaraet al., 2016).
According to the Ministry of Health of Brazil (MHB, 2019), in the first 11 weeks of 2018, 62.900 cases of dengue were registered in this country, and in 2019 this number increased by 264.1%, with a total of 229.064 cases in the same period of the year. Different insecticides (organophosphates and pyrethroids) and larvicides (organophosphates and growth regulators) are used to control A. aegypti in Brazil due to the increasing resistance of this vector (Augusto et al., 2016). However, the use of these products can cause harmful effects on human health and the environment (Mendes et al., 2017).
The increasing resistance of mosquito populations has led to studies on alternative tools for vector control. From this perspective, vegetable compounds are a promising and environmentally safe strategy to prevent larvae survival due to the bioactive properties of their compounds (Marangoniet al., 2012; Garcezet al., 2013).
The effectiveness of the bioactive extracts, oils, and isolated compounds obtained from vegetables are being researched with great efforts, increasing the list of plants with medicinal and pest control properties (Garcezet al., 2013). These plant species are a promising source for vector control due to their low toxicity to humans and other living organisms, low concentrations needed, and no cumulative effect on the environment (Garcezet al., 2013).
Natural compounds with larvicidal or insecticidal effects are an alternative for A.aegypti control since these products are generally less harmful to non-target organisms, biodegradable, efficient, and with low cost (Mendeset al., 2017). The interest in its medicinal uses has attracted the attention from traditional communities (Barroset al., 2014). However, there is a lack of information in the literature about its use as a larvicide. In this context, this study aimed to evaluate the larvicidal activity of vegetable oils from Caryocar coriaceum, Mauritia flexuosa, Carapa guianensis, Copaifera langsdorffii, Ricinus communis and Cocos nucifera against A. aegypti larvae.
MATERIALS AND METHODS
Installation of traps
To obtain A. aegypti eggs, oviposition traps (ovitraps), black plastic pots (400 mL capacity) with 10% hay extract, and pressed wood pallet (type Eucatex) were installed in residences of Crato and Juazeiro do Norte, Ceará, Brazil. The traps were collected 5 days after installation, and straws with the eggs were immersed in tap water for larvae hatching. The hatched larvae remained in the trays with water and were fed with fish food (Alcon Pet, Santa Catarina, Brazil) until the third-instar larval (L3) (Silvaet al., 2017).
Vegetable oils obtaining and application
The vegetable oils were purchased at local family farmers' fairs in the municipalities of Crato and Juazeiro do Norte-CE. The oils were selected according to their medicinal use and their sale frequency at the fairs. C. coriaceum oil is composed of oleic acid (50.2%) and palmitic acid (44.3%), as main components (Croda do Brasil, 2002). M. flexuosa oil has 79.6% oleic acid, 16.1% palmitic acid and 1.3% linoleic acid (Soares et al., 2020). C. guianensis oil has myristic acid (0.04%), linolenic acid (0.2%), behenic acid (0.3%), palmitoleic acid (0.8%), arachidic acid (1.4%), stearic acid (8.9%), linoleic acid (9.5%), palmitic acid (27.7%) and oleic acid (50.9%) (Azevedo et al., 2017). C. langsdorffii oil is composed of 55 to 60% of sesquiterpene acids (Cascon, 2000). R. communis oil is composed of 84 to 91% ricinoleic acid, oleic acid (3.1-5.9%), linoleic (2.9-6.5%), steric (1.4-2.1%) and palmitic (0.9-1.5%) (Embrapa, 2017) and C. nucifera oil is composed of lauric acid (45-53%), myristic (16-21%), palmitic (7-10%), caprylic (5-10%) and capric (5-8%) and the main triacylglycerol is trilaurine (22.2-23.9%), which consists of a glycerol esterified with three lauric acids ( Silva et al., 2020).
The study was carried out at the Agricultural Entomology Laboratory, Center for Agricultural and Biodiversity Sciences, Federal University of Cariri, from May to June of 2019.
A two-step assay was carried out. In the first step, the most toxicologically efficient vegetable oils againstA. aegyptilarvae were selected. In the second step, the lethal doses of the selected oils were determined. The assays were set under environmental conditions, with temperature and relative humidity monitored with a Thermo hygrometer.
First bioassay
A completely randomized experimental design was used with seven treatments: pequi (Caryocar coriaceumWittm.), buriti (Mauritia flexuosaL.), andiroba (Carapa guianensisAubl.), copaiba (Copaifera langsdorffiiDesf.), castor (Ricinus communisL.), and coconut (Cocos nuciferaL.) vegetable oil, and water used as the control treatment, with four replications with 10 larvae at the L3 instar for each replication.
The doses of the treatments used were adjusted at 500 ppm plus Tween® 20 used as a surfactant to aid in the dilution of the oils in water. In the control treatment, distilled water and Tween® 20 were used. The larvae were submitted to the treatments for 24, 48, 72, 96, and 120 h of exposure. Mortality was assessed when dead larvae did not react to the mechanical stimulus of fine-pointed forceps.
Second bioassay
After selection of the oils with the best larvicidal effect againstA. aegypti(oils fromC. coriaceumandM. flexuosa), a second bioassay was set up. A completely randomized experimental design was used in a 2x6 factorial scheme. The treatments consisted of six concentrations for each oil (C. coriaceumandM. flexuosa- 0, 500, 1000, 1500, 2000, and 2500 ppm). The control treatment and assessment of larvae mortality followed the same procedures as described in the first bioassay.
Mortality efficiency
The larvae mortality efficiency was determined using the following equation (Abbott, 1925): E (%)=[((Nc-Nt)/Nc))x100], where, E=efficiency; Nc=number of alive individuals in the control treatment; Nt=number of alive individuals in the treatments.
Statistical analysis
Data were submitted to analysis of variance (ANOVA) using the R software. Means of quantitative factors were compared by the Tukey test, and means of qualitative factors by regression analysis at 5% probability. Probit analysis (Finney, 1971) was performed in the second assay to obtain the LD10, LD50, and LD90 through the PoloPlus 1.0 software, with 5% confidence interval level significance.
RESULTS AND DISCUSSION
After contact ofA. aegyptilarvae with the vegetable oils, their movements became slow, in addition to tremors and convulsions. In tests with organophosphates and plant products against larvae ofA. aegyptiandA. Albopictus, the same symptoms were observed (Kaniset al., 2012). The oil ofM. flexuosacaused the highest mortality of the larvae after 24 h and was only statistically different fromC. langsdorffii. The oilsof C. coriaceum, C. guinanensis, R. communis,andC. nuciferawere statistically equal.The oil of C. langsdorffiicaused the lowest mortality and was statistically different from the other oils. During the other four exposure periods, no statistical difference between the treatments was observed (Table 1). The ethanolic extract ofAzadichta indicaat the dose of 50 mg L-1 caused 93% mortality ofA. aegypti larvae after 72 h of exposure (Manzanoet al., 2020).
Table 1 Mortality (number of dead larvae) and efficiency (%) of Aedes aegyptilarvae submitted to the medicinal oils within each exposure period at the dose of 500 ppm.
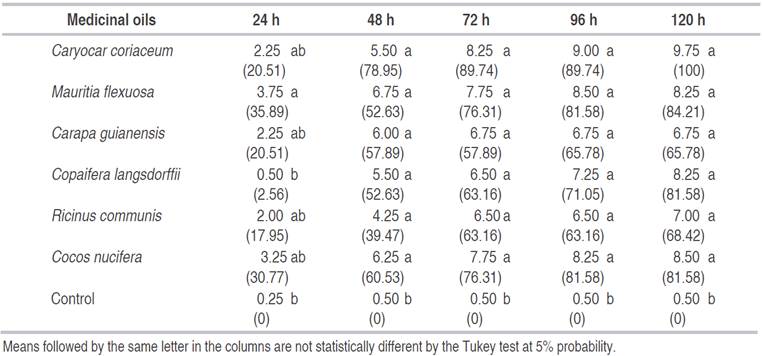
According to Abbot's efficiency test, represented by the values in parentheses, the oil ofM. flexuosawas the most efficient after 24 h of exposure, with almost 36%, followed byC. nuciferaandC. coriaceumwith 30.77 and 20.51%, respectively (Table 1). The oil ofC. nuciferacaused a 50% mortality in A. aegypti larvae under the same conditions and for the same exposure period, at 500 ppm (Fazalet al., 2013).
Only R. communisoil did not surpass 50% mortality after 48 h of exposure. After 96 h the oil of this plant, in a dose of 20% of the product, caused a larvae mortality of 45% (Neveset al., 2014). This oil is mostly composed of 90% ricinoleic acid, and although it causes low mortality inA. aegyptilarvae, it is effective in controlling cashew white fly nymphs (Aleurodicus cocoisCurtis), with an efficiency of over 90% between 48 and 120 h after application, using 2% oil (Silvaet al., 2007).
The C. coriaceum oil provided the best mortality efficiency48 h after exposure, with a 60% increase compared to the previous period. The C. guianensisoil provided the lowest progression in larvae mortality, with the lowest efficiency value compared to the other oils. However, the repellent activity of this oil against adults ofA. aegyptiwas reported (Bueno and Andrade, 2010).
The C. langsdorffiioil caused a 90% mortality 96 h after exposure at 200 ppm (Trindadeet al., 2013). In the present study, mortality of more than 80% occurred 120 h after exposure at 500 ppm. These differences are probably due to the oil purity since some extractors usually dilute the product before sale. The higher nutritional quality and commercial value of authentic vegetable oils have led to their adulteration with the use of low-grade (seed oils), refined pomace, or esterified oils (Popescuet al., 2015). Adulteration in other high-price oils is still the biggest source of agricultural fraud problems (Zhanget al., 2014).
The highest efficiency was obtained by the C. coriaceum oil, followed byM. flexuosaandC. nucifera oils, which provided the same efficiency 72 and 96 h after larvae exposure. After 120 h, onlyC. coriaceumcaused 100% mortality, followed byM. flexuosaoil with 84.21% efficiency. The last two oils were selected for the second assay due to their higher larvae mortality in the dose of 500 ppm, 120 h after exposure. TheC. coriaceumis a plant of the Caryocaraceae family native to the Brazilian Cerrado. Its fruits are very rich in oil, proteins, and carotenoids. The oil, which is composed mainly of unsaturated fatty acids, is considered of great quality.
Fatty acids, carotenoids, and ascorbic acid are found in the chemical composition ofM. flexuosa.
According to the analysis of variance, in the second assay (Table 2), an interaction betweenC. coriaceumandM. flexuosaoils was observed within the doses and the exposure periods. No significant difference between the mortality rates was observed. However significant interaction was obtained between oil and period, and concentration and period.
As observed in the interaction with the oils and exposure time, a significant difference was observed at 5% probability. TheC. coriaceum oil provided the highest means (Table 3). Similar behavior was observed 48 h after exposure of the larvae to the treatments.
Table 3 Larvicidal effect (number of dead larvae) of Caryocar coriaceum and Mauritia flexuosa oils within each exposure periods.

For the remaining exposure periods, no statistical difference was observed between the two treatments. BothC. coriaceumandM. flexuosaoil effectively controlledA. aegyptilarvae when exposed for 72, 96, and 120 h.
The total mean doses of theC. coriaceumandM. flexuosaoils when submitted to regression analysis (Figure 1), provided the effectiveness of the larvicidal effect in relation to exposure time, and at the period of 120 h after exposure, both oils caused higher mortality. Thus, it could be presumed that the 2-degree polynomial regression curves represent well the toxic activity of these oils againstA. aegypti.
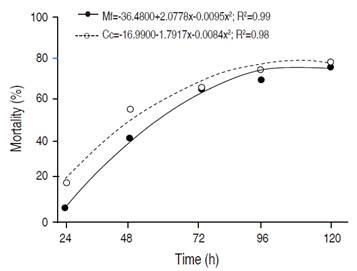
Figure 1 Mortality of Aedes aegypti larvae under application of Caryocar coriaceum and Mauritia flexuosa oils. Mf = Mauritia flexuosa; Cc = Caryocar coriaceum.
According to the interaction analysis between the doses and the exposure time (Table 4), it was observed that regardless of the oil used, the dose of 2500 ppm promoted the highest mortality with 24 h after exposure. The same occurred 48 h after exposure; nevertheless, the doses of 500, 1000, 1500, and 2000 ppm did not statistically differ at 5% probability.
Table 4 Larvae mortality (number of dead larvae) of Aedes aegypti under doses of Caryocar coriaceumand Mauritia flexuosaoils and exposure period.
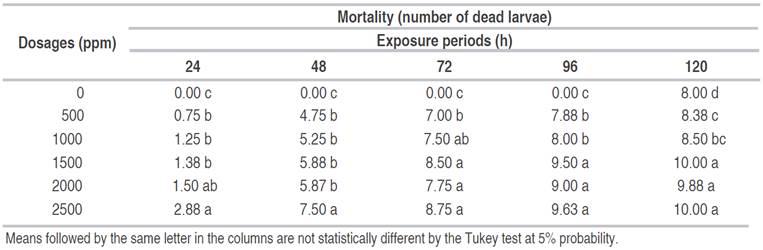
The doses of 2000 and 2500 ppm promoted the highest mortality rates, with no statistical differences when the larvae were exposed for 72, 96, and 120 h. Therefore, the dose increase was proportional to the increase in the number of dead larvae. The dose of 2500 ppm and the exposure period of 120 h were the most appropriate conditions to cause the highest mortality rate ofA. aegyptilarvae.
The larvicidal activity of theC. coriaceumethanolic extract at 250 ppm was evaluated againstA. albopictuslarvae. A mortality of 65 and 95% was observed 24 and 48 h after exposure, respectively. All larvae were dead 24 h after exposure when the extract was used at 1000 ppm (Vianaet al., 2018). In the present study higher doses were required to cause mortality to the A. aegyptilarvae. The larvicidal activity ofNigella sativaoil againstA. aegyptihad LC90 at 523.5 ppm after 12 h of larvae exposure (Rajet al., 2015).
The minimum (LD10), median (LD50), and maximum (LD90) doses ofM. flexuosaoil were able to cause mortality inA. aegyptilarvae (Table 5). It was not possible to perform the Probit test for theC. coriaceumoil treatment due to the lack of variation in mortality within the concentrations since the dose of 500 ppm was enough to kill 100% of the larvae.
A LD50 value of 620 ppm was obtained forArtemisia abrotamumleaf extract, a value relatively close to the value obtained in the present study. However, forCurcuma longaL. andMelaleuca leucadendronL. oils, the LD50 values were lower, 113 and 120 ppm, respectively (Leyvaet al., 2008). Aneeset al. (2008) found that the Ocimum sanctumL. oil had a lethal concentration of 425.94 ppm againstA. aegypti, while for larvaeof Culex quinquefasciatus, the LD50 was 592.60 ppm. Different results were obtained forCroton tiglium,Cascabela thevetia,Ricinus communis, andDatura stramoniumseed oils. The larvicidal activity of this oil againstA. aegyptihad LC50 of 82.08, 95.19, 80.83 and 88.69 ppm, respectively, 24 h after larvae exposure (Borahet al., 2012).
CONCLUSIONS
The medicinalCaryocar coriaceum, Mauritia flexuosa, Carapa guianensis, Copaifera langsdorffii, Ricinus communis, andCocos nuciferaoils have a larvicidal activity on third-instar stage larvae ofAedes aegypti, with greater efficiency 120 h after exposure.
TheCaryocar coriaceumandMauritia flexuosa oilsprovided the highest control efficacy ofAedes aegyptilarvae, at 2500 ppm. The recommended LD10, LD50, and LD90 ofMauritia flexuosaoil to controlAedes aegyptilarvae are 234, 648, and 1794 ppm, respectively.