Tomato (Solanum lycopersicum L.) is the most important fresh and processed vegetable globally (it is also considered as a fruit), and after potatoes, it is the most consumed vegetable depicting 16% of the total consumption (Heuvelink, 2018). In 2019, China (1'086,771 ha; 62.86 million t), India (781,000 ha; 19.0 million t), Turkey (181,488 ha; 12.84 million t), and United States (110,760 ha; 10.85 million t) were the major producers and have remained for several years as the world leaders in tomato cultivation (FAOSTAT, 2019). The world average tomato productivity was about 3.7 kg m-2; nonetheless, the Netherlands stands out as the country with the highest yield (50.7 kg m-2), far from countries considered as main producers, such as China (5.78 kg m-2), India (2.43 kg m-2), Turkey (7.07 kg m-2) and United States (9.8 kg m-2) (FAOSTAT, 2019). In Colombia (18,608 ha; 0.82 million t) the average tomato yield in open-field cultivation is 2.86 kg m-2, while in greenhouse conditions reaches values of 8.3 kg m-2 (Agronet, 2019). However, despite the huge global area cultivated with tomato, the use of grafted plants represents only 0.81% (600 ha) in the United States and a maximum of 1% in China (10,000 ha). By contrast, in countries such as Vietnam, Korea, Japan, France, and the Netherlands, the grafted tomato crop represents between 25 and 75% of the total area (Singh et al., 2017; Singh et al., 2020). According to Lee et al. (2010), the use of grafted plants is a strategy that has been increasing in parallel with the growth of the tomato crop under protected conditions. In its beginnings, the use of grafted materials was done for the prevention of biotic limitations, but nowadays, grafting is considered an effective strategy to improve tolerance of plants to abiotic stresses (Ashok and Sanket, 2017; Gaion et al., 2018; Meimandi and Kappel, 2020; Reddy, 2016; Sen et al., 2018; Singh et al., 2019), such as soil salinization, extreme temperatures and humidity, high or low radiation, water stresses, heavy metals and organic pollutant (Nordey et al., 2020; Rouphael et al., 2017; Singh et al., 2020; Xie et al., 2020; Zhang et al., 2019). Regarding the use of tomato grafted plants, various studies have shown increases in yield compared to non-grafted plants (Grieneisen et al., 2018; Khah et al., 2006; Reddy, 2016; Turhan et al., 2011; Zeist et al., 2017); nonetheless, Bhatt et al. (2015) found no differences between grafted and non-grafted plants, and even Goto et al. (2013) reported that the use of graft plants has a negative effect on tomato crop yield.
Various studies have been carried out to determine the effect of this relationship on the grafted plants growth, development, biomass production, and photosynthetic activity (Martínez-Ballesta et al., 2010). Bhatt et al. (2015) observed an increase in photosynthetic activity (23%) in young tomato grafted plants (60 to 70 days after transplanting - dat) relating to self-grafted and non-grafted plants. In comparison, He et al. (2009) indicated that no significant differences in photosynthetic activity were found for the same combination of plants, with values between 23 and 25 μmol CO2 m-2 s-1. Similar results were obtained by Zhang and Guo (2019), who determined that photosynthesis in tomato scion grafted to a potato rootstock was similar to tomato seed plants (14.4 to 16.4 μmol CO2 m-2 s-1). On the other hand, few studies have been carried out in adult plants (>70 dat). Fullana-Pericàs et al. (2018) measured gas exchange (100 dat) in tomato plants grafted in "Maxifort" commercial rootstock showed significantly higher net photosynthesis (33 μmol CO2 m-2 s-1) than non-grafted (25 μmol CO2 m-2 s-1) and grafted on 'Beaufort' rootstock (27 μmol CO2 m-2 s-1). Nonetheless, there is no consensus about the benefits of grafting tomato, despite the variety of research carried out worldwide; the results have not been conclusive, ranging from a positive physiology effect and production parameters to adverse outcomes, for that reason, "the use of grafting affects the physiology of the tomato plant and increase fruit yield becoming a good strategy for the Colombian tomato producer" was stated as hypotheses; keeping this in view, the present work aimed to evaluate the physiological response of a commercial tomato scion, grafted on different rootstocks in conditions of the high-Andean region in Colombia.
MATERIALS AND METHODS
Location: the present experiment was carried out under plastic house conditions, at the municipality of El Santuario, Antioquia, Colombia (6°6'55.8"N and 75°13'10.15"W, an altitude of 2,251 m), which is localized in the high-Andean region. A sandy loam-clay-sandy textural class soil was used in the experiment, pH (5.0), EC (0.06 dS·m-1), soil organic matter (5.8%), phosphorus (66 mg·kg-1 soil), sulfur (53.2 mg·kg-1 soil), Ca (10.6 cmolc kg-1), Mg (3.0 cmolc·kg-1), K (2.47 cmolc kg-1), ECEC (16.5 cmolc kg-1), Fe (74 mg kg-1), Mn (9 mg kg-1), Cu (9 mg kg-1), Zn (5 mg kg-1) and B (0.2 mg kg-1). During the tomato growth period (April 29 to October 25, 2019), with a portable thermohygrometer (Extech RHT20), the climatic variables recorded inside the plastic house were minimum, mean, and maximum temperature (°C) and relative humidity (%).
Experimental design: a randomized complete block experimental design was used, with four (4) replication and four (4) treatments. The treatments consisted of a single commercial tomato scion grafted on different rootstocks combination: vigor rootstock (VR), resistant rootstock (RR), self-grafting plants (SELF), and plants no grafted (Seedlings).
Plant material: the genotype (S. lycopresicum L.) used as a scion was a tomato cv. Libertador, and as a rootstock, two commercial materials were used: "Olimpo" as vigorous rootstock, and "Armada" as resistant rootstock to Ralstonia solanacearum and Fusarium oxysporum f. sp. radicis-lycopersici. The grafting method applied was the tongue approach grafting, described by Lee et al. (2010). The Seedlings and grafted plants were transplanted to the plastic house on April 29, 2019, each of them, with the third leaf on the main shoot folded, corresponding to 103 stages according to BBCH scale proposed by Feller et al. (1997). The field planting distance was 1.1 m between rows and 0.45 m between plants, and as part of the management, the first lateral shoot was allowed to grow below the first inflorescence to have two stems per plant, for a density of 20,200 plants ha-1 and 40,400 stems ha-1. The growth of the plants was allowed until the ninth fruit cluster's emission on the main stem and seventh fruit cluster on the lateral stem, for a total of 16 fruit clusters emitted throughout the life cycle.
Gas exchange parameters: according to the BBCH scale in the 701 (first fruit cluster), 704 (fourth fruit cluster), 706 (sixth fruit cluster), 708 (eighth fruit cluster), 710 (tenth fruit cluster), and 712 (twelfth fruit cluster) phenological stages, with the portable infrared gas analyzer LCi - ADC (Bioscience, UK), provided by an external halogen lamp ADC (Bioscience, UK) gas-exchange measurements were done within the youngest completely expanded leaf closes to each fruit cluster, under a saturating photosynthetic photon flux density (PPFD) of 1,100 μmol m−2 s−1. Net photosynthesis (A, μmol CO2 m-2 s-1), stomatal conductance (gs, mol H2O m-2 s-1) and transpiration rate (E, mmol H2O m-2 s-1) were measured between 9:00 am and 11:00 am. The water-use efficiency (WUE) was calculated as the ratio between the net CO2 assimilation rate (A) and the transpiration rate (μmol CO2 mmol H2O-1), and radiation use efficiency (RUE) as the ratio A/PPFD (μmol CO2·μmol photon-1).
Chlorophyll fluorescence: it was measured in the same leaf used to determine the gas exchange parameters at the same phenological stages (except 706). Measurements were performed with a portable fluorimeter Opti-Sciences (Opti-Sciences Inc., Tyngsboro, MA, EEUU). The measurements were taken on dark-adapted leaves for 30 min before each reading began, and daily measurements of quantum yield of PSII- Qy (Fv/Fm) were made.
Leaf area index (LAI): it was measured in the same tomato phenological stages used to determine the chlorophyll fluorescence parameters through a 'SunScan' Canopy Analysis System System SS1 (Delta-T, UK).
Dry matter and tomato yield: at the end of the crop cycle, the remaining leaves of each plant were harvested, and the dry matter was determined. For this, the fresh biomass was dried in an oven (Memmert UL 80) at 60 °C, until a constant weight was reached. Harvest index (HI) was determined by the proportion of dry biomass of tomato in relation to total dry biomass. Tomato yield was estimated as the sum of the total weight of 16 fruit clusters harvested per plant (kg plant-1). The harvest started at 89 dat (07/21/2019) and ended at 188 dat (10/28/2019).
Statistical analysis: one-way ANOVA analyzed the variances among different treatments, and their multiple comparisons were analyzed by Tukey's HSD (Honestly significant difference) test, P<0.05. When the data did not meet the assumptions of normality, additivity and homogeneity of variance, the Kruskal-Wallis test, and the mean comparison (LSD, P<0.05) were applied using the R project "agricolae" package.
RESULTS AND DISCUSSION
The mean temperature into plastic house fluctuated between 20 and 25 °C, within the optimal range (18 to 25 °C) to develop the crop (Camejo et al., 2005; Heuvelink, 2018). On the other hand, 30 to 40 °C was the maximum temperature reached, and the minimum temperature was between 10 to 15 °C (Figure 1). Regarding relative humidity, mean daily values between 70 and 90% were recorded, which, as indicated by Jaramillo-Noreña et al. (2012), would be above the optimal mean daily values from 50 to 65% of this climatic variable for the crop (Figure 1).
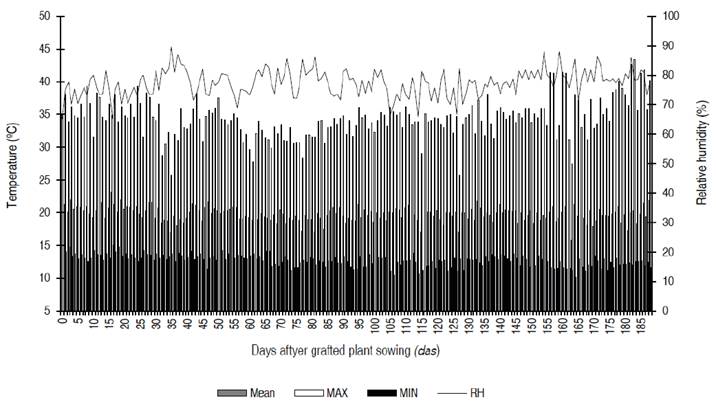
Figure 1 Daily mean (Mean), maximum (MAX) and minimum (MIN) temperature and relative humidity (RH) into plastic house between April 23 and October 25, 2019.
Gas exchange: the highest photosynthesis rate (A) was recorded in the initial stages of the production phase (701), reaching values of 26 to 32 µmol CO2 m-2 s-1, about the final phase (712), where photosynthetic activity declined on average by 73% (Table 1). At the initial stages (plants 37 dat) of development (701), no significant differences were found between the treatments due to scion×rootstock combinations; nevertheless, in more advanced stages of development 704 (fourth fruit cluster), the use of resistant rootstocks (RR) and self-graft (SELF) reached the highest A values, which showed significant differences respect to the vigor rootstock (VR) and non-grafted plants (Seedling) (Table 1).
Table 1 Evaluated parameters among different scion×rootstock combinations in different tomato phenological states according to the BBCH scale (701, 704, 706, 708, 710 and 712).
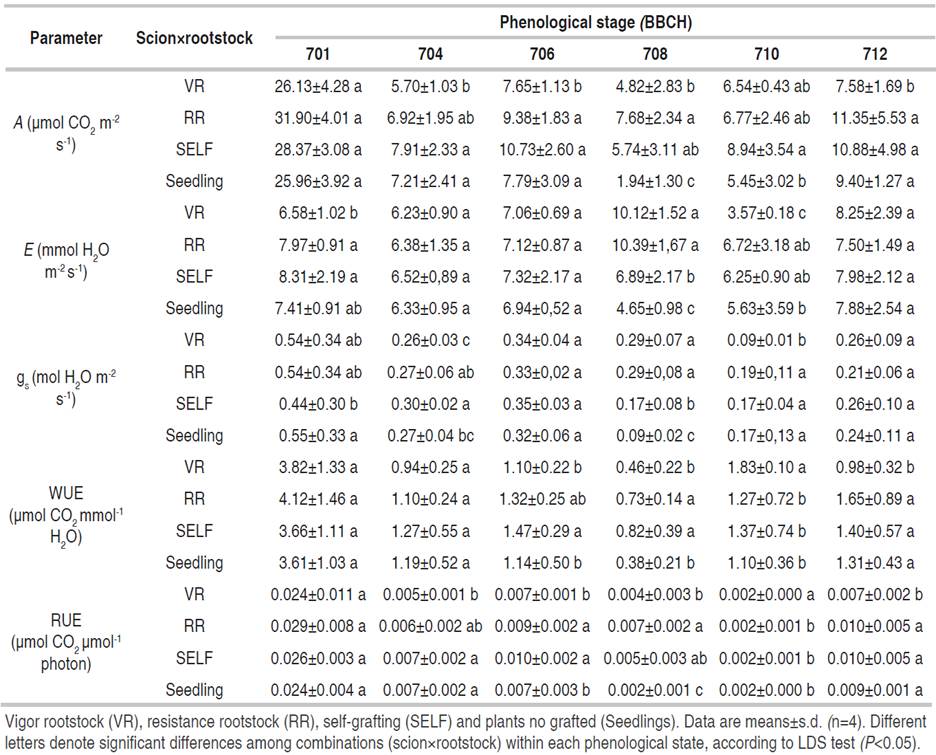
Large differences between the initial phenological stages (701) and the other ones may indicate that the tomato in its initial stages becomes a strong source because this part of the cycle begins processes of floral differentiation followed by fruit formation; likewise, the emission of lateral branches occurs and the leaf area increases, thus, the photosynthetic activity decreases.
The A values for young plants (701 - 37 dat) are considered high, since the values reported by Bhatt et al. (2015) for scion×rootstock combinations do not exceed a fixation rate of 20 µmol CO2 m-2 s-1 and, even according to Camejo et al. (2005) and Ogweno et al. (2008) not grafted plants (60 growing days) reached 10 µmol CO2 m-2 s-1 of A at constant PPFD of 350 μmol photons m−2 s-1 and 20 µmol CO2 m-2 s-1 to 800 μmol photons m−2 s−1 of radiation. On the other hand, Khan et al. (2019) in non-grafted plants determined an increase in photosynthetic activity with age, A values of 8 and 13 µmol CO2 m-2 s-1, with a PPFD of 800 μmol photons m−2 s−1, were observed for plants with 60 and 90 dat respectively; which was different from that observed in the present study, where plants in advanced phenological states, recorded less than 10 µmol CO2 m-2 s-1 for A values.
Both plants, at the beginning and end of development, non-statistical differences were observed in transpiration rate (E), with an average of 7 mmol H2O m-2 s-1, except to phenological stage 708, where the VR and RR treatments showed an increase 78% of E compared to self-grafted and non-grafted plants of the same age (Table 1).
Similar to photosynthesis, stomatal conductance (gs) was higher in both grafted and non-grafted plants, at the beginning (701) of the productive stage, compared to those in more advanced production states (710), where gs declined on average by 53% (Table 1). In the 701 phenological stage, significant differences were observed with a reduction of gs by 19%, in self-graft plats, concerning the other two combinations of scion×rootstock (VR and RR) and plants from seed (Seedling) (Table 1). Although the higher gs (0.54 mol H2O m-2 s-1) was achieved at the start of production (701) in the VR, RR, and SELF-treatments, these observed values are lower than those reported in other works, where gs varied between 0.6 a 0.8 mol H2O m-2 s-1 (Camejo et al., 2005; Khan et al., 2019; Ogweno et al., 2008). On the other hand, higher gs values were reported by Bhatt et al. (2015) in young tomato plants grafted, self-grafted, and non-grafted, where stomatal conductance between 0.2 and 0.25 mol H2O m-2 s-1 was reached.
All scion×rootstock combinations make young tomato plants more efficient, WUE and RUE showed values of 4 μmol CO2 mmol-1 H2O and 0.025 mmol CO2 µmol-1 photons. Nevertheless, a decrease of WUE (70%) and RUE (77%) was observed at the final phenological stages (Table 1). In general, no relevant result for WUE and RUE was observed for any scion×rootstock combination through different phenological stages. Bhatt et al. (2015) showed WUE values for young grafted and non-grafted tomato plants of 3.2 to 3.4 μmol CO2 mmol-1 H2O, and for plants in the productive stage of 1.1 μmol CO2 mmol-1 H2O, comparable to those reported by Khan et al. (2019). According to some authors, the RUE for tomato varies from 0.0141 to 0.0286 mmol CO2·µmol-1 photons, being similar to the efficiency between tomato plants in the initial and final stages of the productive cycle (Bhatt et al., 2015; Camejo et al., 2005; Khan et al., 2019; Ogweno et al., 2008).
Chlorophyll fIuorescence: the quantum yield (Qy) of photosystem II (PSII) did not differ significantly through the different phenological stages (701 to 712) of the tomato according to BBCH scale, for scion×rootstock combinations evaluated (Figure 2). The Qy values varied from 0.74 to 0.79, according to various authors, these values are considered as normal in healthy plants and without stress, which depending on the genotype, can vary between 0.69 and 0.80 (Bhatt et al., 2015; Goto et al., 2013; Zhou et al., 2018).
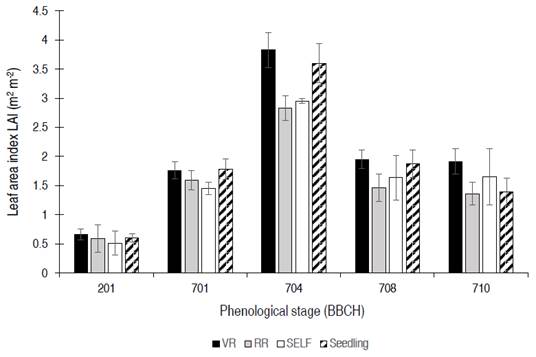
Figure 2 Quantum yield (Qy) among the different scion×rootstock combinations: vigor rootstock (VR), resistance rootstock (RR). Self-grafting (SELF) and plants no grafted (Seedlings), in different tomato phenological states (701, 704, 708, 710 and 712) according to the BBCH scale. Data are means±s.d. (n=4) and denote significant differences among combinations within each phenological state, according to LDS test (P<0.05).
Calatayud et al. (2013) considered Qy as a practical and direct parameter to determine incompatibility between rootstocks and scion. On the other hand, Martínez-Ballesta et al. (2010) affirm that the incompatibility of the graft can become evident in the early stages, when vascular connections are formed, or also, in the fruiting stage, when the plant has a high demand for water and nutrients. Nevertheless, in this study, as shown in Figure 2, the Qy of PSII was always in values that indicated physiological health and the absence of post-graft stress.
Leaf area index: when tomato plants reached their highest leaf development (704), significant differences were found between scion×rootstock combinations. The highest LAI was obtained by VR (3.8) compared to RR (2.8) and SELF (2.95), but similar to Seedling (3.6), indicating that the rootstock can modify the physiological components of yield in tomato, such as LAI (Figure 3). According to Heuvelink (2018), the optimal LAI for tomato plants is 4 to 5, values that were achieved for VR treatment at 704 phenological stage (3.8). From this moment of cultivation, it was necessary to carry out pruning to form the plant architecture and eliminate lateral shoots and diseased branches and leaves, thus, from this phenological stage (704), was evident an LAI reduction, with values remaining between 1.5 to 2.0, without significant differences between scion×rootstock combinations.
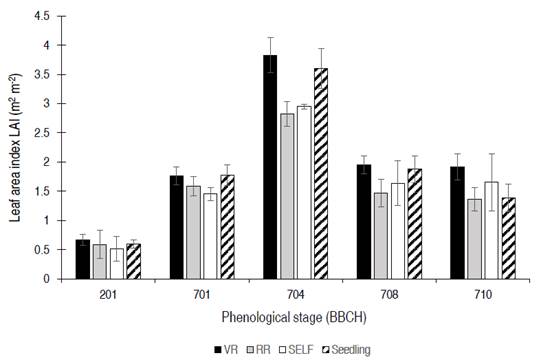
Figure 3 Leaf area index (LAI) among the different scion×rootstock combinations: vigor rootstock (VR), resistance rootstock (RR). Self-grafting (SELF) and plants no grafted (Seedlings), in different tomato phenological states (701, 704, 708, 710 and 712) according to the BBCH scale. Data are means±s.d. (n=4) and denote significant differences among combinations within each phenological stage, according to Honestly Significant Difference - HSD test (P <0.05).
Dry matter: the scion×rootstock combination that generated the greatest accumulation of dry biomass in leaves, fruits, and the whole tomato plant was the VR, differing significantly from the other combinations. VR accumulated 44% more leaf biomass and 28% more dry biomass in the entire plant, which can be related positively to the highest LAI reached at 704 phenological stage by RV (Figure 3, Table 2). However, although authors such as Nilsen et al. (2014) affirmed that the callus formed by the scion×rootstock union can reduce not only the flow of water to the shoots but also the leaf area and limits the transport of photoassimilates to the roots; this results in a slower growth of grafted plants than the non-grafted ones. In this study, the results did not show a negative effect leaf development by the scion×rootstock combinations evaluated (Figure 3, Table 2), the use of a rootstock with vigor characteristics (VR) represented a significant gain in the total dry matter of leaves and fruits in relation to the plants grafted in resistant rootstock (RR), self-grafted (SELF) and plants from seed (Figure 3); similar to that exposed by Calatayud et al. (2013) who indicate that leaf weight and leaf area are directly correlated, the higher the weight, the greater the leaf area.
Table 2 Dry matter, harvest index (HI) and tomato yield among the different scion×rootstock combinations in different tomato phenological states (701, 704, 706, 708, 710 and 712) according to the BBCH scale.
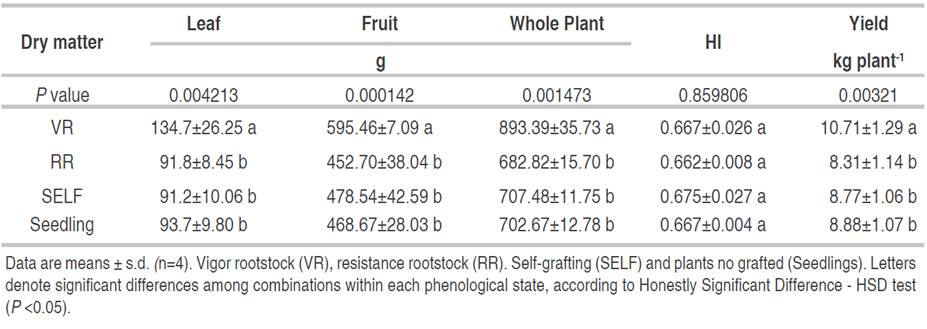
An interesting relationship between the LAI and leaf dry matter values is proposed in the plants grafted on VR rootstock. The higher LAI observed on VR plants at 704 before pruning allows a higher photosynthesis in the whole canopy. In this sense, increases in photosynthesis and carbohydrate export from leaves to fruits should increase tomato fruit growth at the end of the cycle shown for VR (Lemoine et al., 2013).
Tomato fruit yield and harvest index: regarding the HI, there was no significant difference; that is, the proportion of biomass distributed towards tomato fruits was not affected by the scion×rootstock combination. However, a significant effect on tomato fruit yield was observed. VR was the scion×rootstock combination that showed the highest yield (kg tomato plant-1), which differed significantly by 22% more in yield, concerning RR, SELF, and Seedling treatments did not present statistical differences among them (Table 2). These results are opposite to the VR combinations but similar to RR and SELF combinations reported by Goto et al. (2013). These authors determined that grafting decreased the dry matter in leaves, the total yield of fruits compared to non-grafted plants. The grafting practice by itself did not increase the yields, as was seen in the self-grafting treatment, which did not have significant difference with the non-grafted plants (Table 2). This result is consistent with that reported by Grieneisen et al. (2018), who did not find significant differences in tomato yield between self-grafted and non-grafted plants, unlike the increase in tomato yield (37%) obtained when hetero grafted plants were used (rootstocks genetically different).
Nevertheless, according to Grieneisen et al. (2018), grafting as an agronomic strategy will not always be associated with a gain in yield; in some cases, the rootstock confers other characteristics, such as resistance to biotic stress and tolerance to abiotic stresses, without a significant increase in the scion fruit yield. To sum up, the yield parameter is the most important when deciding which scion × combination to choose. The other parameters will help to understand what makes one treatment the same or different from another.
CONCLUSION
Net photosynthesis, stomatal conductance, efficient use of water, and efficient use of radiation were higher in the initial stages of the productive stage. The quantum efficiency of the PSII did not show evidence of any stress due to grafting, since the values were those characteristics of plants with adequate functioning. Physiological parameters of the commercial scion when grafted on a vigorous rootstock were lower than those of the other scion×rootstock combinations; however, the yield, dry matter and LAI had an opposite behavior. Despite vigor rootstock presented the lowest values of A, it was the one that showed the greatest leaf, fruit, and whole plant dry matter accumulation, and the highest LAI, resulting in higher production of tomato fruit.