In Mexico, tomato production is increasing. In 2017, the harvested volume was more than 631 thousand tons. Tomato is in the first place of exported agricultural produce (SIAP, 2018). However, safety specifications for fresh produce can put this progressive preference for the vegetable at risk because production practices and post-harvest handling conditions occur in environments that favor the development of pathogenic and deteriorative microorganisms (Orozco et al., 2008).
Firmness and color are the most significant quality attributes of fresh tomatoes used by growers and consumers as selection criteria (Khairi et al., 2015), while the content of sugars and organic acids are important factors that determine flavor (Agius et al., 2018). During post-harvest, these attributes are affected by storage conditions, such as temperature and relative humidity. Low temperatures can substantially increase shelf life by slowing down the fruit ripening process and reducing microbial activity (Guatam et al., 2015). A wide variety of microbial species can lodge on the surface of the product adhering and forming biofilms, rendering the washing and disinfection processes ineffective (Iturriaga et al., 2003). The chance of bacterial contamination of fruits is high, given that the content of water and nutrients support bacterial growth, besides micro-rough texture facilitating bacterial adhesion and establishment (Torres-Aguilar et al., 2016).
Adherence, as a survival mechanism, allows enteropathogens of the genera Escherichia, Salmonella, and Shigella to remain on tomatoes, lettuce, peach, spinach, broccoli, alfalfa, and apple and orange juice, whose consumption is associated with outbreaks of gastrointestinal disease (Scallan et al., 2011). This becomes even more important when these fresh vegetables are eaten raw in salads or juices. In developing countries, enterotoxigenic Escherichia coli (ETEC) is the second pathogroup that causes gastroenteritis in children during their first years of life (Walker et al., 2007). ETEC is also linked to traveler’s diarrhea caused by lack of hygiene and fecal contamination (Loc et al., 2014). ETEC’s virulence factors are the heat-labile toxin and the heat-stable toxin (Fleckenstein et al., 2010). Furthermore, these bacteria can survive in a variety of environments, such as rivers, drinking water, irrigation water, and fresh vegetables (MacDonald et al., 2015).
There are several studies in tomatoes concerning E. coli contamination during production, harvest, and commercialization (Gómez-Aldapa et al., 2013). However, there are few references on the relation of quality attributes and survival of E. coli on fruits stored at different temperatures. For this reason, this study aimed to evaluate the effect of 7 and 22 °C temperatures for 168 h of storage on tomatoes inoculated with enterotoxigenic E. coli (ETEC) as well as color indexes (hue angle, h°, and chroma, C*), firmness, titratable acidity (% citric acid), ascorbic acid, total soluble sugars and reducing sugars (glucose, fructose, and sucrose).
MATERIALS AND METHODS
Plant material
The material used consisted of fruits of tomato (Solanum lycopersicum L.) Charleston cv. harvested at 5 degrees (luminous red), according to the color table of the Department of Agriculture of the United States (USDA, 2017), and collected from the central part of the hydroponic type greenhouse in Aquixtla, Puebla, Mexico. The fruits were transported and maintained in refrigeration in a cooler for less than 12 h before laboratory analyses started.
Studied microorganism
The enterotoxigenic E. coli (ETEC) pathogroup strain was isolated from tomato fruits and the soil of the greenhouse (Luna-Guevara et al., 2012). This strain was identified by IMViC biochemical tests (indol, methyl red, Voges-Proskauer, and Simmons citrate) and tests of the automated system VITEK (Biomeriux, Mexico) and confirmed by polymerase chain reaction (PCR) with amplification of the IngA gene (Luna-Guevara et al., 2015).
Fruit inoculation and storage procedures
Intact fruits were washed and disinfected with a 70% (v/v) ethanol solution, rinsed with sterile water, and dried. Fruits were inoculated by immersion for 10 minutes in a 107 CFU mL-1 bacterial culture with an optical density (OD) 1.1 at a wavelength of 620 nm. Inoculation was confirmed by plate count. The inoculated fruits were deposited in plastic hermetically sealed containers (25x10x20 cm) and stored at two temperatures (T): 7 and 22 °C with an interior relative humidity (RH) of 60% adjusted with saturated sodium bromide salts (Iturriaga et al., 2007). Interior T and RH of the containers were monitored with an environment datalogger (HOBO H08-004-02, Onset Pro Computer Corporation, MA, USA).
Microbial counts
Approximately, 2 mm of tissue was taken from the epicarp and mesocarp at the equatorial region of the fruit. This tissue was homogenized in 50 mL of 0.1% peptone water. Serial dilutions (104 to 107) were made and spread on plates in trypticase soy agar (Bioxon, Mexico) for enumeration of E. coli. The plates were incubated at 37 °C for 24 h and the presence of E. coli was confirmed using conventional IMViC biochemical tests. This procedure was repeated in triplicate at 1.5, 24, 72, 120, and 168 h of storage.
Observation of adherence and colonization
Micrographs of the inoculated fruits stored for 1.5 up to 168 h were prepared for examination with a scanning electron microscopy (SEM) following the procedure proposed by Sun et al. (2016) with some modifications. The samples were observed in a scanning electron microscope (JEOL, JSM-6390, MA, USA) 10-15 kV range of operation.
Physical tests on fruits stored at different temperatures
Color. The color parameters CIE L*, a*, and b* were determined in triplicate at the equatorial zone of five fruits using a colorimeter (Hunterlab, ColorFlex-45) (Pathare et al., 2013). With these chromatids, hue angle (h°, related to reds and greens) and color purity (C*, chroma) were calculated.
Firmness. The firmness of intact fruits was determined as the force required for a 6 mm diameter cylindrical TA-212 awl and a texturometer TAXT plus (Texture Technologies, Surrey, UK) to penetrate 5 mm at a velocity of 1.0 mm s-1. The results were expressed in Newtons (N) and each reported value of firmness represents the mean of three individual measurements taken on three tomato samples.
Titratable acidity. The acidity of the juice extracted from 10 g of fruit was evaluated by titration with NaOH at 0.1 N until reaching a pH of 8.1. The result was expressed as a percentage of citric acid (% citric acid) (Horwitz, 2000).
Chemical properties of fruits stored at different temperatures
Total soluble (TSS) and reducing sugars (RS).
For both types of sugars, 100 mg of sample was extracted and incubated in periods of 10 min five times successively in 80% ethanol at 70 °C. The supernatants were evaporated at 50 °C, dissolved in 1 mL of distilled water, and stored at -20 °C until analysis. The TSS were determined following the Antrona method proposed by Montreuil et al. (1997) and RS were quantified using the method described by Scholes et al. (1994). The calculations used standard calibration curves, which were prepared previously for each of the sugars, results were expressed in g ∙100 g-1 fresh weight (f.w.).
Vitamin C. Total ascorbic acid was analyzed by the spectrophotometer method described by Noctor and Foyer (1998) using 100 mg fruit. Absorbance readings were carried out in a spectrophotometer UV/Vis (JEYWAY 7305, ThermoLab, USA) at a wavelength of 265 nm before and after adding 20 µL ascorbate oxidase (0.05 U). The blank consisted of a 120 mM sodium phosphate buffer, pH 5.6.
Statistical analysis
The experimental data were analyzed statistically by a completely randomized experimental design with three replications. For the set of treatments, an analysis of variance (ANOVA) was performed and means were compared with an honest significant difference of P<0.05 (Tukey). The Statistical Analysis System (SAS), version 9.0 (SAS, 2002) was used.
RESULTS AND DISCUSSION
Effects of storage conditions on Escherichia coli (ETEC) growth
Effect of storage conditions on E. coli (ETEC) survival on inoculated tomato fruit was significant (P<0.05), the bacterial populations were 7 and 9.2 Log CFU g-1 at 7 and 22 °C, respectively, after 120 h of storage. This behavior is similar to that reported by Gómez-Aldapa et al. (2013), who reported the growth of E. coli (enterotoxigenic) in mung bean sprouts and the growth of E. coli on foods stored in refrigeration (Kothe et al., 2019).
The E. coli (ETEC) counts of CFU g-1 recorded at refrigeration temperature (Figure 1) evidence the psychrotrophic capacity of this microorganism to grow in fresh food products (Pothakos et al., 2012; Keshri et al., 2019), and its permanence under this condition indicates that it favors ETEC survival. For this reason, the consumption of fresh tomatoes makes it necessary to consider preventive measures to maintain the safety of the fruit and avoid it from becoming a reservoir of enterobacteria, including E. coli, which can produce gastrointestinal disorders (Mansan-Almeida et al., 2013).
Adherence of ETEC on tomatoes stored at different temperatures
The pericarp of fruits stored at 7 and 22 °C showed growth of ETEC from 1.5 h of storage (Figures 2A and 2B, respectively). After 72 h post-inoculation, adherence of E. coli was notable (Figures 2C and 2D). According to Shaw et al. (2011), adherence is carried out through a diffuse mechanism of adhesion mediated mainly by adhesins of the flagella on vegetables. Other reports have shown that E. coli can produce biofilms through curli and extracellular matrix (1.5-n-acetyl-D-glucosaminecellulose, cellulose, and colonic acid) on sprouts and tomato roots (Matthysse et al., 2008). It has been observed that the growth of enteric pathogens such as ETEC is greater in plant tissue with mechanical damage due to the availability of nutrients (Shaw et al., 2011).
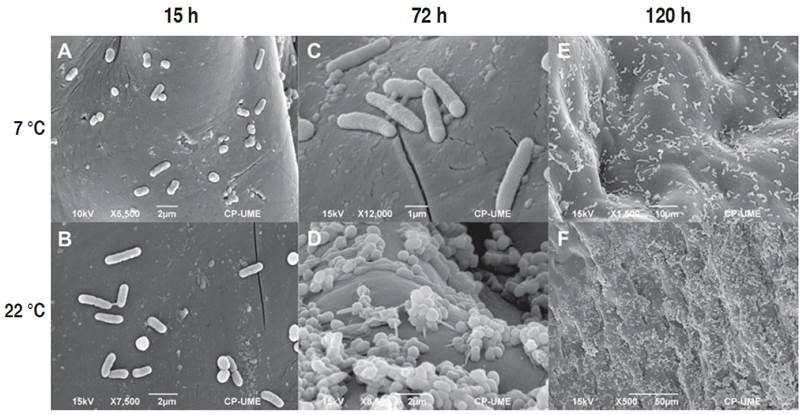
Figure 2 Micrographs of tomato inoculated with Escherichia coli (ETEC) at 7 and 22 °C. Micrographs after 1.5 h (A, B), 72 h (C, D), and 168 h (E, F) of storage.
Adhesion of the bacteria on the fruit surface under both storage conditions persisted after 168 h is shown in Figures 2E and 2F. The greatest adherence of ETEC at 22 °C (Figure 2E) was related to 120 h of storage (Figure 1). The presence of E coli. strains that can form biofilms in both conditions suggests potential health risk for consumers (Liu et al., 2013; Corzo-Ariyama et al., 2019), given that this contamination may take place during the pre-harvest period, due to the use of a contaminated water supply when cultivating the vegetables, in post-harvest environments, where it may appear after washing and processing the raw material, also due to storage temperatures which allow fast growth of the bacterial (Carter et al., 2016).
Effect of storage conditions on color and firmness parameters
Color components of inoculated fruits were affected significantly (P<0.05) by storage temperature. The chroma (C*) value increased as the fruit ripened and showed an increase in color intensity over time. Similar results were described by Navarro-López et al. (2012). There was a greater increase in fruits stored at 22 °C, which retained their red color (Table 1). According to López-Camelo and Gómez (2004), the value of C* influences consumer acceptance of ripe fruits, and thus, inoculated fruits stored at room temperature and in refrigeration are acceptable for the consumer based on color intensity after 168 h. Hue angle, h°, decreased with storage time at both temperatures. The fruits stored at 7 °C tended to change color less and had significantly higher values than fruits stored at 22 °C. The decrease in h° of fruits stored at 22 °C was more notable at 72 h (Table 1), and even after 168 h, the hue angle of the fruits (24.69) decreased 2.6 times, regarding fruits stored under refrigeration (64.32). The results obtained in this index suggest that the fruits stored at room temperature (22 °C) had a greater color change, this is due to the fact that the tomato fruit being climacteric, their physiological processes continue after harvest and as their maturation progresses, chlorophyll degradation occurs, as well as chromoplast synthesis, promoting the color change from green to red, which shows the presence of pigments such as carotene and lycopene (Pinheiro et al., 2013; Carrillo-López and Yahia, 2014; Cherono et al., 2018).
Table 1 Physicochemical properties in tomato inoculated with Escherichia coli (ETEC), stored at 7 and 22 °C.
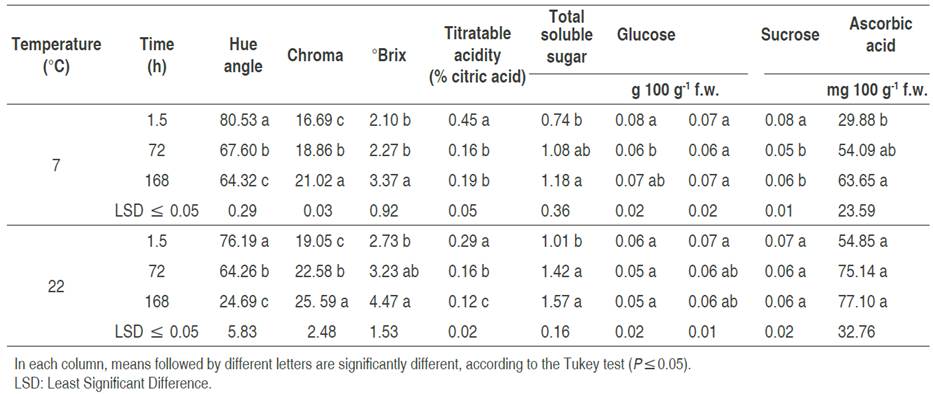
Firmness tended to decrease under both conditions of storage temperature, but at 22 °C it was significantly less (P<0.05) up to 168 h (Figure 3). Room temperature can cause a continuous reduction in tomato firmness due to moisture loss through transpiration and enzymatic changes, which can degrade tomato cell wall (Hatami et al., 2013; Abiso et al., 2015). Firmness reduction is attributed to different factors as losses in cell turgor pressure as well as the cell wall and polysaccharides degradation (Al-Dairi et al., 2021). The tendency of the experimental fruit firmness values at 7 °C is similar to that mentioned by Tadesse et al. (2015) and Kabir et al. (2020), who stated that tomato fruits are better preserved in refrigeration at 4 °C because the low temperatures maintain the quality characteristics of the tomato in postharvest.
Effect of storage conditions on organic acids and concentration of total soluble and reducing sugars
Citric acid is the most abundant acid in tomatoes and the largest contributor to titratable acidity. The decrease of acidity coincides with the increase in fruit ripening and is due to the loss of citric acid (Anthon et al., 2011). In this study, this parameter decreased significantly (P<0.05) as the ripening process advanced; the decrease was more notable in fruits stored at 22 °C (Table 1). In tomato, the ascorbic acid contents are higher in stage full maturation (De Oliveira et al., 2016), which is consistent with the results of this study that although no differences were detected in the three evaluated stages, the highest values were detected at 22 °C, where the fruit maturation occurs more quickly. While at 7 °C, a significant increase (P<0.05) was observed up to 168 h (Table 1).
Alenazi et al. (2020) pointed out that the content of total soluble sugars is related to tomato maturity. In this investigation, the total soluble sugars increased as the fruits ripened, and this was more accentuated in fruits stored at 22 °C than in those stored at 7 °C. In both conditions, significant differences were detected (P<0.05) at 72 and 168 h. According to Oms-Oliu et al. (2011), tomato fruit maturation involves changes in its physiology, for example, the increase in sugars, such as glucose and fructose, and sucrose reduction. This trend was more marked in the total soluble sugars in the fruits stored at 22 °C after 72 h.
The conservation of sugars in tomato fruits under refrigeration at 7 °C was not significantly different. This is similar to that reported by Buret et al. (1983), who confirmed that the sugar content in tomato fruits is constant at low storage temperatures. At 22 °C fruits were significantly different in sugar content at some times, with an increase at 162 h. Fructose had statistically equal values in fruits stored at 7 and 22 °C, with a significant difference at the longest storage times (72 and 168 h). The fructose contents, associated with the decrease of some organic acids, contribute directly to the flavor of ripe tomatoes (De Oliveira et al., 2016). Finally, sucrose showed a slight increase after 168 h at 7 °C, while at 22 °C there was no significant difference with constant and minor values (Table 1). In this work, approximately equal amounts of the three sugars (glucose, fructose, and sucrose) were detected in the analyzed fruits regardless of the storage temperature.
CONCLUSIONS
Storage conditions significantly affected tomato fruits. ETEC survived with populations of 7 and 9.2 Log CFU g-1 at 7 and 22 °C, respectively, after 120 h of storage. The adherence and bacterial colonization in storage were confirmed by scanning electron microscopy. At 22 °C, glucose, sucrose, h° parameters, and firmness were significantly more affected than at 7 °C. The concentration of fructose did not exhibit a significant difference at 7 °C.
The permanence of ETEC in tomatoes stored at 22 °C makes it necessary to propose strategies of sanitization process to minimize conditions of contamination and preserve the quality parameters of the tomato, during post-harvest storage to maintain the fruit’s safety and prevent it from serving as a reservoir of enterobacteria capable of producing gastrointestinal disorders.