Vacuum impregnation is a technique that allows the introduction of food ingredients directly into the product, through its pores, in a controlled way, according to the model of the hydrodynamic mechanism (Andrés et al., 2001; Radziejewska et al., 2014; Zhao and Xie, 2004; Nawirska-Olszańska et al., 2020; Derossi et al., 2021). The potential of this technology to enhance the physical-chemical characteristics, sensory attributes, or health potential of the product depends on the selection of appropriate processing conditions, such as vacuum pressure (VP) and osmotic solution (OS) (Fito et al., 1996). Pressure produces significant changes in the product structure altering the fresh-like quality. Macroscopic properties (optical or mechanical properties) are modified and consequently, the related appearance and textural attributes also (Zhao and Xie, 2004; Cortez-Latorre et al., 2021). Assis et al. (2019) and Mejía-Águila et al. (2021) worked with apples at 50 mbar and demonstrated the suitability of mild VP. Practically, all the native liquid was removed from the pore structure using VP<400 mbar (Zhao and Xie, 2004). OS composition is also important; for instance, treatments based on citric acid (CA) and ascorbic acid (AA) avoid or reduce enzymatic browning. Honey is a natural anti-browning agent due to its antioxidant activity, which is attributed to the vitamin C, small peptides, flavonoids, and other phenolic compounds, enzymes (glucose oxidase and catalase), and low pH (Jeon and Zhao, 2005). Honey is also a source of antioxidants that reduce the risk of heart disease, cancer, immune system deficiency, cataracts, different inflammatory processes, etc (Boussaid et al., 2018). Concentration of OS is also important. Mujica-Paz et al. (2003) found that OS<50 °Brix impregnated massively in the fruit, however, OS>50°Brix are highly viscous and difficult to penetrate the pores of the plant tissue. Regarding storage temperature, Pirovani et al. (2015) reported that fresh-cut fruit shelf life is longer stored at 0 °C than those stored at 5 to 10 °C. Cortez-Latorre et al. (2021) found increases in vitamin C content, total phenolic content, and antioxidant activity for vacuum impregnated fresh-cut apples storage at 1.5 °C for 7 days.
The high porosity of apple tissue allows vacuum impregnation with hypertonic solutions. In this sense, vacuum and relaxation times are essential processing variables. During the vacuum time, the occluded gas inside the food pores expanded and flows outside the tissue; during the relaxation time, the external OS flows into the pores. Vacuum and relaxation periods can be set in order to attain the desired goals, such as high solids impregnation or high sample dehydration (Paes et al., 2007; Cortez-Latorre et al., 2021).
The objective of the present work was to select the adequate OS based on honey, (alone or with the addition of citric and ascorbic acids) to improve the quality attributes of fresh-cut apples by mild vacuum impregnation.
MATERIALS AND METHODS
Fresh-cut apple processing
Apples (Granny Smith cv.) were purchased from a local market and stored at 1.5 °C until processing. Fruits were selected, washed, peeled, cored, and cut into cubes of 1.5 cm side. Vacuum impregnation was carried out using vacuum laboratory equipment composed of a glass vacuum desiccator (6 L volume) connected to a vacuum pump (Bombacio 31 042/169).
The fresh-cut apple processing conditions were selected according to preliminary studies (Faicán et al., 2018), The vacuum impregnation treatments were performed at 22 °C and 67.7 mbar of VP with a weight ratio of 1:10 fruit: OS, during 14 min of vacuum time (VT) and 7.5 min of relaxation time (RT). Three OSs were used: M0: 30 °Brix honey solution (HS); M0.5: HS+0.5% CA+0.5% AA; and M1: HS+1% CA+1% AA. Finally, apple cubes were placed on a mesh for 1 min to allow OS to be drained from the surface and then on absorbent paper for removal of excess OS. Then, 200 g of apple cubes were packed in 0.42 mm thick PET plastic containers, with a surface area of 0.045 m2, with permeability for O2 = 1.62x101 mL mil cm-2 hr-1 atm-1, and for CO2=3.68x101 mL mil cm-2 hr-1 atm-1 (Exama et al., 1993). Samples were analyzed on the day of vacuum impregnation processing (day 0) and after 7 days of storage at 1.5 °C (day 7).
Mass transfer parameters: Solid gain (SG), water loss (WL), and weight reduction (WR)
The mass transfer parameters SG, WL, and WR were determined based on Equations 1, 2, and 3, respectively,
according to da Conceição Silva et al. (2012).
Where: Mi=fresh-cut fruit weight (g); Mf=vacuum impregnated fresh-cut fruit weight (g); Hi=fresh-cut fruit moisture content (%); Hf=vacuum impregnated fresh-cut fruit moisture content (%). Determinations were done in triplicate.
Negative values for SG, WL, and WR indicate solid loss, water gain, and an increase in sample weight, respectively.
Soluble solids, pH, and moisture content
To determine soluble solids (SS), pH, and moisture content, samples were crushed in a homogenizer. SS were measured with a PAL-ALFA digital portable refractometer (Atago, Tokyo, Japan). A Cardy Twin B-113 pH meter (Horiba Ltd. Kyoto, Japan) was used for pH determination. Moisture content was determined using a PMR50 moisture analyzer (RADWAG. Poland). Determinations were done in triplicate.
Firmness
Firmness was evaluated using TA. XT Plus texture analyzer (Stable Micro Systems, UK) according to Cortez-Latorre et al. (2021). Penetration tests were performed using a cylindrical tip 11 mm long and 4 mm in diameter, with a 50 N load cell. The penetration distance was 8 mm; the test speed was 1 mm s-1. Exponent software was used to determine the maximum force (F) expressed in Newton (N). The F value represents the maximum force exerted by the tip to penetrate each cube. Then, 10 individual cubes were measured, and the mean values of those measurements are reported.
Color
Color was measured with a Minolta CM 508-d spectrophotometer, using an observer angle of 10° illuminant D65, and specular component excluded. L*, a*, b*, hab, C*ab, and the total color difference (∆E*ab) were the color parameters evaluated. Luminosity varies from L*=100 (white) to L*=0 (black); the parameter Cab* indicates the chromaticity or saturation: hab is the hue angle, 0° (red), 90° (yellow), 180° (green), and 270° (blue). In total, 10 individual cubes were measured, and the mean value of those measurements was reported (Piagentini and Pirovani, 2017). Total color difference (∆E*ab) is defined by equation 4:
Where:
δL*i = L*TFi-L*FF
δa*i = a*TFi-a*FF
δb*i = b*TFi-b*FF
Where: FF=fresh-cut fruit, TF=vacuum impregnated fresh-cut fruit, and i=storage day: day 0 or day 7.
Vitamin C
First, 100 g of sample was crushed, and then 12 g was homogenized for 1 min with 25 mL of extraction solvent (30 g L-1 metaphosphoric acid and 80 g L-1 acetic acid), sonicated for 15 min, and then centrifuged at 4000 g at 4 °C for 20 min. Supernatants were separated and used for vitamin C analysis (Rodríguez-Arzuaga et al., 2021). Aliquots of supernatant were pre-treated with DL-dithiothreitol (DTT) solution (0.005 g L-1 prepared in 2.58 mol L-1 potassium phosphate dibasic). Extractions were made in duplicate. Total ascorbic acid content was determined by HPLC according to Van de Velde et al. (2012). Determinations were performed in triplicate. Results were expressed as mg kg-1 of fresh weight.
Total phenolic compounds content
Apple samples (100 g) were crushed in a homogenizer. A mixture of 2.5 g was homogenized with 25 mL extraction solvent (acetone/water 80:20), sonicated for 15 min, and then centrifuged at 4000 g for 20 min at 4 °C. Supernatants were separated and used for the analysis, the determination was performed using the Folin-Ciocalteu reagent, according to Cortez et al. (2018). Determinations were performed in triplicate. Results were expressed as mg GAE Kg-1of fresh weight.
Antioxidant capacity
Apple cubes (100 g) were crushed in a homogenizer. A mixture of 2.5 g was homogenized with 25 mL extraction solvent (acetone/water 80:20), sonicated for 15 min, and then centrifuged at 4000 g for 20 min at 4 °C. The supernatants were separated and used for analysis. Antioxidant capacity (AC) was measured according to Sánchez-Moreno et al. (2003). The anti-radical activity was quantified by measuring the decrease in absorbance of a methanolic solution of the free radical DPPH* (1,1-dipheny-2-picrylhydrazyl) at 517 nm in the presence of aliquots of fruit extracts. Determinations were done in triplicate. Results were expressed as mg AA Kg-1 of fresh weight.
Statistical analysis
Results for soluble solids, pH, firmness, color parameters (except ∆E*ab), total phenolic compounds, vitamin C and antioxidant capacity are expressed as a relative variation percentage for each attribute Q (ΔQi, %), with respect to fresh-cut fruit attribute (Equation 5):
Where: QFF= fresh-cut fruit attribute, QTF=vacuum impregnated fresh-cut fruit attribute, and i= storage time: day 0 or day 7.
Data were analyzed using ANOVA. The differences among mean values were determined by the Tukey test at P≤0.05. Statistical analyses were carried out using STATGRAPHICS Centurion XV 15.2.06 (Statpoint Technologies, Inc., Warrenton, VA, USA).
RESULTS AND DISCUSSION
Mass transfer parameters: Solid gain (SG), water loss (WL), and weight reduction (WR)
Table 1 shows the mass transfer parameters in fresh-cut apples subjected to mild vacuum impregnation. There were no significant changes for SG among OSs (P≥0.05). The average SG was 2.8%.
The SG found in the present work was higher than the SG value obtained for fresh-cut apples impregnated at 67.7 mbar with VT=10 min, RT=10 min, and 30 °Brix sucrose OS (2.4%) reported by Faicán et al. (2018). This discrepant result may be attributed to the difference in molecular weight between solutes of honey (glucose, fructose, maltose, and other sugars that correspond to approximately 80% of honey composition) and those of sucrose. Bolin et al. (1983) found different responses of fresh-cut apples, peaches, and apricots subjected to osmotic dehydration with two OSs, sucrose and high fructose corn syrup, with the latter causing a greater increase in SG. According to those authors, sucrose, a disaccharide, would be expected to migrate slower than fructose, a monosaccharide. The diffusion coefficient of fructose is 32% higher than that of sucrose, consequently, honey OS may have penetrated faster replacing more of the water in the cells. Zhao and Xie (2004) suggested that in most cases, low molecular weight carbohydrates are used in impregnation treatments since this condition allows a faster penetration into the sample. These results are consistent with the findings in the present study, because honey OS penetrated better than sucrose solution and, consequently, SG was higher.
Table 1 shows that OS affected WL (P≤0.05). No differences between M0 and M0.5 treatments were detected; however, M1 treatment produced greater WL. WR was also affected by OS (P≤0.05). All treatments resulted in a weight gain (indicated by the negative sign of WR). The present results agree with those of Mujica-Paz et al. (2003), who reported similar results for fresh-cut Golden Delicious apples due to the high porosity of apples, which facilitates the impregnation of hypertonic solutions (40 °Brix).
Physico-chemical parameters
The soluble solids (SS), pH, firmness, color parameters (L*. hab, C*ab), Total Ascorbic Acid (TAA), Total Phenolic Content (TPC) and Antioxidant Capacity (AC) for fresh-cut fruit are presented in Table 2.
Table 3 shows the relative variation percentage for SS as a function of OS on days 0 (∆SS0) and 7 of storage at 1.5 °C (∆SS7). No significant effect of OS (P≥0.05) was observed on either day. SS increased in VI fresh-cut fruit with respect to fresh-cut fruit by approximately 25% and 24%, on days 0 and 7 of storage at 1.5 °C, respectively.
Table 3 Relative variation percentage of soluble solids (∆SSi), pH (∆pHi), and firmness (∆Fi) in vacuum impregnated fresh-cut apples on days 0 and 7 of storage at 1.5 °C.
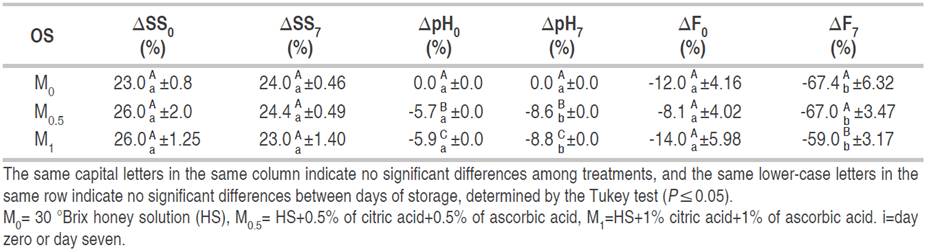
There were significant differences in SS between days 0 and 7 for M0 treatment (P≤0.05) (Table 3). Conversely, M0.5 and M1 treatments did not show differences between days 0 and 7 of storage at 1.5 °C. Granny Smith fresh-cut apples impregnated with 50 °Brix honey OS, 700 mbar, VT=10 min, and RT=10 min showed an increase of SS of about 50-70%, remaining stable after 7 days of storage (Rößle et al., 2011). They found good adherence of honey OS to fresh-cut fruit, which allows the values to remain stable during storage. Similar results in the M0.5 and M1 treatments.
The ∆pH0 and ∆pH7 were affected by OS (P≤0.05). The application of M0 treatment did not cause changes in pH. However, pH values decreased in M0.5 and M1 treatments, due to the presence of AA and CA in OSs (Table 2). The present results are consistent with those of Faicán et al. (2018), who reported variations in VI fresh-cut Granny Smith apples under different VPs and treatment times (VP=67.7 and 667.3 mbar, VT/RT=3 min; VT/RT=10 min), with 30 °Brix sucrose OS with the addition of 1% of AA and 1% of CA; the authors obtained negative values of ΔpH, which means a reduction in pH for VI fruits.
No significant differences in pH between days 0 and 7 were observed in M0 treatment. Nevertheless, for M0.5 and M1 treatments, the ∆pH on day 7 was 1.5 times higher than on day 0. These results agree with those of Cortez-Latorre et al. (2021), who reported a decrease in pH, both on days 0 and 7 (7.7 and 5.1%, respectively) for VI fresh-cut apples with an OS of sucrose with an addition of 1% AA and 1% CA, under the same pressure and time conditions as in the present work.
The relative variation percentage of firmness (∆Fi) was not affected by the OS (P≥0.05) on day 0 (Table 3). The mean percentage was -11.4%. On the other hand, the firmness after 7 days of storage was influenced by OS used in the vacuum impregnation procedure (P≤0.05). M0 and M0.5 treatments had a higher reduction in firmness than M1 (Table 3).
The present results agree with those obtained by Rößle et al. (2011), who reported firmness losses of about 20% on day 0 and higher on day 7 (approximately 25%) in vacuum impregnated Granny Smith apples (VT=10 min and VP=700 mbar) with honey OS. This behavior could be due to VT and OS concentration conditions.
According to Rößle et al. (2011), the firmness of fresh-cut apples impregnated with high-fructose corn syrup (50%) was significantly reduced, whereas OS with lower concentration (20%) did not show firmness reduction. Zhao and Xie (2004) suggested that with the use of hypertonic solutions, the cells may shrink or shrivel due to water leaving the cell. Hence, the selection of impregnation solutions depends on the purpose of osmotic treatment, i.e., the type of finished product, because the type of OS significantly affects mass transfer during the vacuum impregnation processing, and product deformation and shrinkage might occur.
Andrés-Bello et al. (2013) studied the effect of acidification (pH=6.2; 4.4 and 3.9) followed by blanching on the firmness of carrot tissue. Blanching at pH 6.2 caused a firmness reduction (≈70%), and acidification with consequent blanching at pH 4.4 increased the firmness (≈50%). However, blanching and acidifying the tissue to pH 3.9 reduce firmness in the same proportion as that obtained using pH=6.2. Degradation of carrot tissue firmness during heat treatment occurred by two different mechanisms, one at neutral pH and another at pH 3.9 since degradation was much lower at an intermediate pH. The results of the present research agree with the behavior reported by Andrés-Bello et al. (2013); indeed, the pH reduction can be one of the reasons for the loss of firmness, since the pH values obtained after the vacuum impregnation process were lower than 3.4. All treatments reduced firmness, with the reduction being higher after seven days. These reductions imply pH values of 3.2 and 3.1.
There were significant differences in firmness between days 0 and 7 (P≤0.05). The relative variation percentage of firmness on day 7 was approximately six to five times higher than on day 0 (Table 4). Texture breakdown of minimally processed tissue is expected to occur as a response to a wound-induced increase in enzymes targeting cell walls and membranes in response to injury. Huber et al. (2001) found that the firmness of light-processed papaya fruit declined more rapidly and extensively than tissue derived from intact fruit stored under identical conditions. In the present work, the processing and storage of VI fresh-cut apples generated tissue degradation, as indicated by Huber et al. (2001). Vacuum impregnation with hypertonic solutions generates firmness reduction due to osmotic dehydration of the product with simultaneous loss of turgor and elasticity that alters cellular resistance. The increase in its viscous character generates changes in the air and liquid fractions of the product and also in its shape and size. Loss of turgor pressure is due to plasmolysis or rupture of the tonoplast and plasmalemma of plant cells. The loss of elasticity is due to air-liquid exchange during vacuum operations (Alzamora et al., 1997).
Table 4 Relative variation percentage of color parameters (∆L*i, ∆habi and ∆C*abi) in vacuum impregnated fresh-cut apples on days 0 and 7 of storage at 1.5 °C
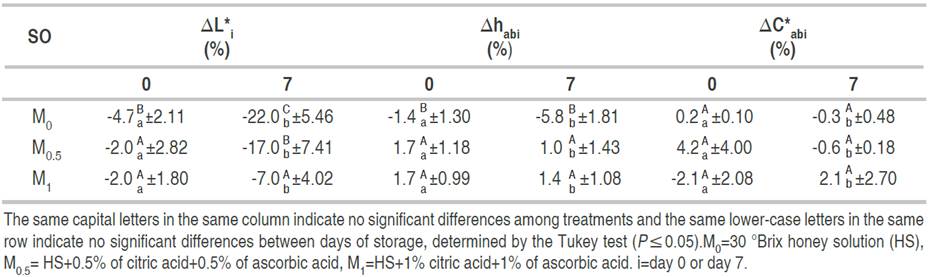
Negative values for the L* relative variation percentage indicate a decrease of the parameter with respect to fresh-cut apples (that is, a darkening of the sample). Positive and negative values in hab relative variation percentage indicate that the hue of vacuum-impregnated fresh-cut fruits was yellower or redder than fresh-cut fruits, respectively. Positive and negative values in C*ab relative variation percentage showed that the chroma of vacuum-impregnated fresh-cut fruits was more vivid or duller than that of fresh-cut fruits, respectively (Table 4).
On day 0, changes in ∆L*0 and ∆hab0 were related to the OS (P≤0.05). However, ∆C*ab0 was not affected by OS (P≥0.05). The ∆L*0 was always negative, meaning that VI fresh-cut fruits were darker than fresh-cut fruits, probably due to honey color. Small changes in ∆L*0 occurred in M0.5 and M1 treatments containing CA and AA (Table 5). The negative value of ∆hab in M0 treatment indicated that vacuum-impregnated fresh-cut apples were redder than untreated fresh-cut ones. On the contrary, M0.5 and M1 treatments resulted in yellower vacuum-impregnated fresh-cut apples. The ∆C*ab0 resulted in an average value of 0.8%. After 7 days of storage, ∆L*7 and ∆hab7 were also affected by the OS used for vacuum impregnation (P≤0.05), but the ∆C*ab7 was not, with a mean value of 0.4% (small changes with respect to fresh-cut fruit). As expected, the lowest changes of ∆L*7 occurred in the M1 treatment due to the antioxidant solution. The comparison of color changes (∆L*, ∆hab, and ∆C*ab), for the same treatment, between days, showed significant differences (P≤0.05) (Table 5).
Table 5 Non-treated fresh-cut apples (FF) and fresh-cut apples were subjected to vacuum impregnation with different osmotic solutions at day 0 and after 7 days of storage at 1.5°C
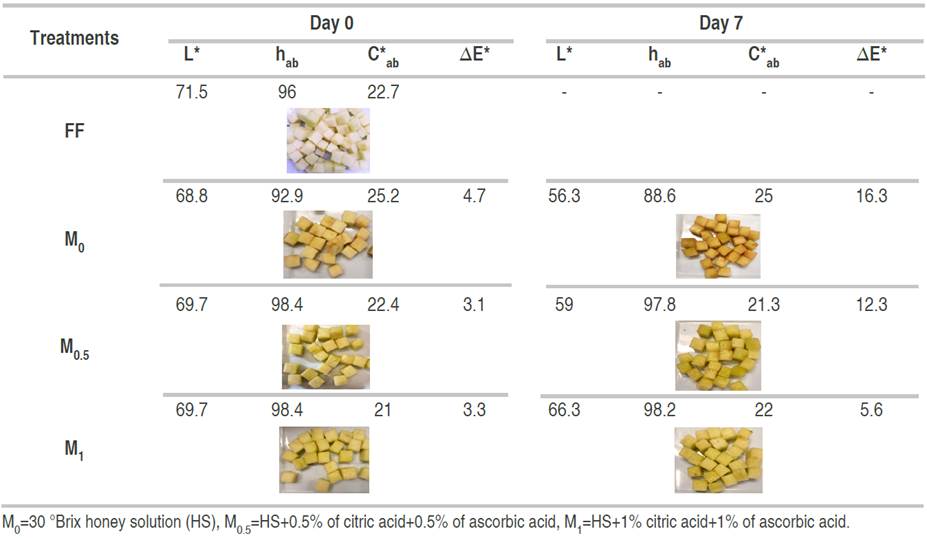
Our results agree with the behavior reported by Jeon and Zhao (2005), who observed that the use of dark honey generates a reduction of L*. This is a potential problem in light-colored fruits such as apples because the fruit acquires a color similar to that of honey. Browning inhibition in crushed Red Delicious apples with the addition of 1% honey and 0.1% AA is three times higher than in samples obtained only with honey addition (Chen et al. 2000).
The total color difference was affected by the OSs on day 0 (∆E*ab 0) and on day 7 (∆E*ab 7) (P≤0.05) (Figure 1). There was a perceptible color difference for M0 samples with respect to untreated fresh-cut apples and fairly perceptible color differences for M0.5 and M1 on day 0. After 7 days, ∆E*ab 7 values showed strong differences for M0 and M0.5 treatments, but only perceptible differences for M1 treatment, which had the highest content of AA and CA. In this sense, it is well known that the use of antioxidant compounds contributes to the maintenance of visual attributes, preventing discoloration due to enzymatic browning (Betoret et al., 2015; Rodríguez-Arzuaga and Piagentini, 2018).
Bioactive compounds
The relative variation percentage of total ascorbic acid content (∆TAAi) on day 0 was significantly affected by the OS (P≤0.05). Treatments M0.5 and M1 increased the vitamin C content with respect to fresh-cut fruits on day 0 Treatment M1 produced a greater increase in total ascorbic acid, 31 times higher than in fresh-cut fruits due to the AA solution. (Figure 2).
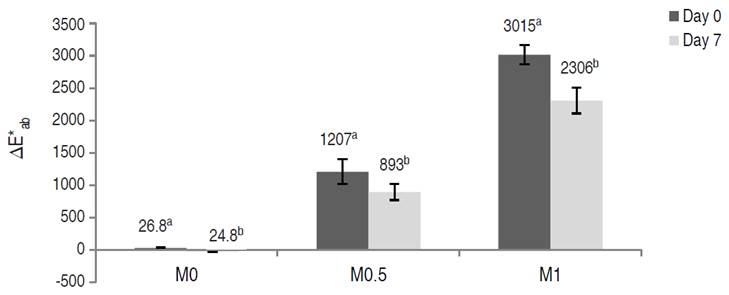
Figure 2 Total ascorbic acid variation (∆TAA) in vacuum-impregnated fresh-cut apples on days 0 and 7 of storage at 1.5 °C.
Cortez-Latorre et al. (2021) studied the effect of vacuum impregnation process variables on quality aspects of fresh-cut apple vacuum-impregnated with OS of sucrose with the addition of 1% AA and 1% CA (VP = 67.7 mbar). They determined a high increase (about 5600%) in total ascorbic acid content of treated fruit with respect to fresh fruit when applying the same VT and RT as in the present study. Here, total ascorbic acid content was reduced in all samples during storage (Figure 2). However, the total ascorbic acid content for M0.5 and M1 was still higher than untreated fresh-cut apples at the end of storage. Rodríguez-Arzuaga et al. (2021) reported that vitamin C content (197 mg kg-1) of Granny Smith apples treated by immersion (3 min) in a solution with 1.2% of Yerba Mate+0.9 % CA+1% AA at atmospheric pressure increased on day 1 and then decreased up to approximately 50 mg kg-1 after 10 days, remaining constant until the end of storage at 2 °C for 18 days and 10 °C for 15 days. Similarly, Cortez-Latorre et al. (2021) determined that fresh-cut apples vacuum-impregnated under the same pressure conditions as those in the present work, with a sucrose OS (30 °Brix) with 1% CA and 1% AA, with VT and RT among 1 to 14 min, maintained high values of vitamin C (188-606 mg kg-1) at the end of 7 days of storage at 1.5 °C.
No significant effect of OS on ∆TPC0 was found (P≥0.05). However, all the vacuum-impregnated samples increased the phenolic compound content with respect to fresh-cut fruit on day 0 (Figure 3). On the other hand, ∆TPC7 was affected by OS (P≤0.05); M0 treatment lost the phenolic compounds (approximately 19%). Treatment M0.5 maintained phenolic compounds similar to fresh-cut apples, and M1 maintained phenolic compounds uptake (about 20%).
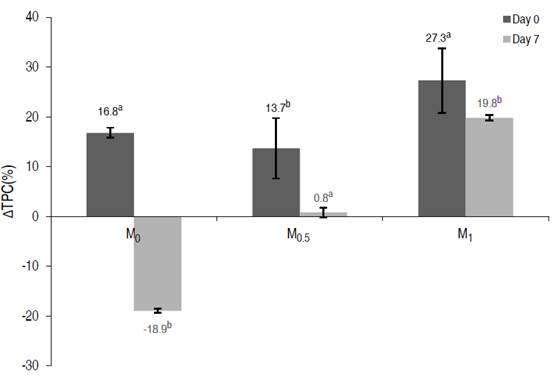
Figure 3 Total phenolic content (∆TPC) in vacuum impregnated fresh-cut apples on days 0 and 7 of storage at 1.5 °C
Oszmianski and Lee (1990) suggested that honey not only inhibits the enzymatic oxidation of polyphenols but also converts part of o-quinones to the original phenols. It is also known that this kind of reaction occurs with AA, which turns the o-quinones into phenols (Robards et al., 1999), and can be the reason for the increase of phenolic compound content after VI treatments in the present study. Nawirska-Olszańska et al. (2020) also evaluated the application of vacuum impregnation technology using apple-pear juice as OS in chokeberry fruit as a treatment before the drying process and determined an increase in the phenolic compound content (46.3-63.9%) after the vacuum impregnation process; the phenolic compounds increase obtained herein shows similar behavior.
On the other hand, the phenolic compound content decreased with M0 treatment without AA and CA after the storage. In this sense, the decrease in polyphenol concentration due to browning was found to be correlated with the degree of enzymatic browning (Murata et al., 1995). Similarly, in this work, apples treated with M0 showed a high color change after 7 days of storage, showing a pronounced browning. The absence of AA and CA in M0 reduces the possibility of delaying enzymatic browning, causing a decrease in phenolic compounds. Like other factors, pH also plays a significant role in the browning of fruits and vegetables. Polyphenol oxidase enzyme activity is inhibited in the presence of acids (Singh et al., 2018). This situation can explain the significant differences in total phenolic content between days 0 and 7 (P≤0.05) for M0 treatment, with increase of phenolic compounds on day 0 due to honey and losses on day 7 of storage due to enzymatic degradation.
The relative variation percentage of antioxidant capacity (∆ACi) was influenced by the OS (P≤0.05). On day 0, the M1 treatment generated the highest increase of ∆AC0. On day 7, M0.5 and M1 generated similar increases, which were higher than in M0 (Figure 4). Similar results were obtained on day 7, with the increase in antioxidant capacity caused by M1 and M0.5 being approximately two times higher than fresh cut apples.
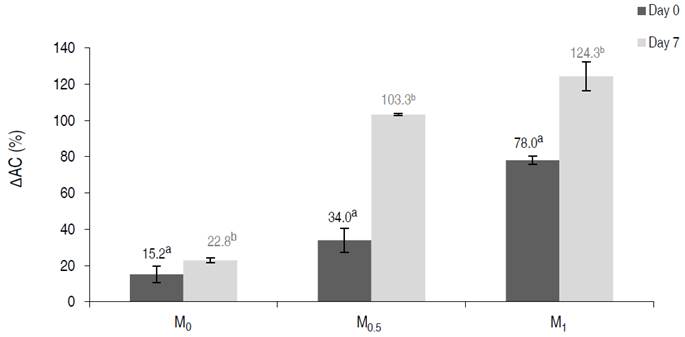
Figure 4 Antioxidant capacity (∆AC) in vacuum impregnated fresh-cut apples on days 0 and 7 of storage at 1.5 °C.
Santarelli et al. (2020) evaluated the effect of frozen storage on the content of functional compounds and the AC of vacuum-impregnated apples treated with an organic lemon juice solution with a citric acid content of 0.5% w/v. They found that vacuum impregnation significantly affects the AC of apple products, leading to increases of 10-13%, and attribute this result to the apple uptake of lemon juice and its antioxidant compounds (e.g., flavonoids, citric acid). Similarly, the present work shows that the small AC increase (15 - 23%) in M0 was due to honey bioactive compounds, whereas higher increases in AC of M0.5 and M1 were related to the uptake of ascorbic and citric acid solution.
Significant differences in ∆AC were observed between days 0 and 7 for treatments M0.5 and M1 (P≤0.05). On day 7, ∆AC increased 3 and 1.6 times in M0.5 and M1 samples, respectively, with respect to day 0. Rodríguez-Arzuaga et al. (2021) studied fresh-cut Granny Smith apples by immersion in a solution containing 1.2% of Yerba Mate + 0.9 % CA+ 1% AA at atmospheric pressure and reported an increase in antioxidant activity during the first two days at 2 °C. This antioxidant activity remained stable and was higher than values in the samples without immersion in antioxidant solution until day 7. Similarly, the capacity antioxidant found in the present study increased when using OS with the addition of CA and AA, after 7 days at 1.5 °C.
CONCLUSION
The use of different OSs affected the final quality of the vacuum-impregnated fresh-cut apples. The most adequate treatment was M1 (30 °Brix honey with 1% AA plus 1% CA), which showed significant increases in vitamin C content, phenolic compound content, and antioxidant capacity. Regarding other quality attributes, there was a slight increase in the soluble solids, a small reduction in firmness, and a fairly perceptible color difference. After 7 days of storage, an additional reduction of firmness was recorded, with the other attributes being maintained or enhanced. The M1 treatment was found to be an appropriate alternative for processing fresh-cut apples with improved healthy potential attributes.