Introduction
Mesophotic coral ecosystems (MCEs) consist of light-dependent corals usually found 30 m deep from the euphotic zone limit where the light incidence is less than 10%. They are very rich and biodiverse in terms of benthic habitats and constitute communities with unique structures (Kahng, et al., 2014; Laverick, et al., 2017; Enrichetti, et al., 2019). MCEs have shown ecological connectivity as source and receptors of fish larvae from shallow zones during or after perturbations and they act as refuge for a high number of species playing an essential role in the physical and biological interactions of the trophic structure (Semmler, et al., 2017; Shlesinger, et al., 2018). This makes them of interest for research and conservation activities.
MCEs species have adapted to low photosynthetically active radiation. Lesser, et al. (2009), Bessell-Browne, et al. (2014), and Nir, et al. (2014) have suggested that some mesophotic corals change their trophic strategy increasing heterotrophy to counteract the reduction of calcification resulting from the zooxanthellae's low photosynthetic rate due to low availability of light energy. Zooplankton becomes the primary food resource in these ecosystems and, therefore, key for the energy transference within MCEs and to understand the ecological processes that allow the existence of such unique environments.
Zooplankton is formed by a large assemblage of heterotrophic organisms with limited movement capability essential for the maintenance of most marine ecosystems, as a link between primary production and multiple trophic levels, and as part of the biogeochemical cycles and benthic and pelagic recruitment, which explains their great trophic importance (Carrillo-Baltodano & Morales-Ramírez, 2016; Nakajima, et al., 2017; MacKenzie, et al., 2019). These organisms have a wide distribution range in world oceans and belong to a great phyla variety of filter feeders with omnivore habits that can be found in different stages of their life cycles (Baéz-Polo, 2013).
Most of the studies on MCEs have focused on scleractinian corals, octocorals, sponges, and fishes (Kahng, et aL, 2014; Scott & Pawlik, 2019) and not so much on zooplankton organisms. Andradi-Brown, et al. (2016) recently reported the first comparisons between zooplankton communities associated with mesophotic and shallow reefs, and they mentioned the need for further research to determine the deep gradient pattern of zooplankton communities and their feeding ecology essential to maintaining MCEs.
The "Corales de Profundidad" National Natural Park (PNNCPR) is the first marine deep zone protected area in the country (Alonso, et al., 2015) whose priority focuses on the study and monitoring of zooplankton given its ecological importance. Our purpose here was to characterize and quantify for the first time the zooplanktonic organisms associated with MCEs in Bajo Fríjol, Colombian Caribbean.
Materials and methods
Study area
The "Corales de Profundidad" National Natural Park (PNNCPR), with an extension of 142.192 km2 and a depth ranging from 34 to 1240 m, is located in the Colombian Caribbean across from the San Bernardo archipelago on the Sinú fold belt tectonic area formed by the interaction of the Caribbean and the South America oceanic plates (Morales, et al., 2017). As our study area inside this marine protected area, we chose the Bajo Fríjol seamount (Figure 1).
Bajo Fríjol is located at the edge of the Colombian Caribbean continental shelf; it has a mostly flat bottom covered mainly by calcareous rhodoliths. It is part of an extension of the relief defining the Tortuga bank and the Barú peninsula whose origin is related with the Colombian Caribbean margin (Morales, et al., 2017). It is an ellipsoidal formation with a long axis of 8.2 km in the northeast-southwest direction and a short axis of 1.4 km. The relief corresponds to a bank with an elevated platform at 116 m between depths of 34 to 150 m, and the top is a sloping terrace (Morales, et al., 2017). The shallowest depth is composed of some coral colonies and hard coralline landforms that adjoin with flat zones a little deeper showing some isolated coral and sponge promontories. The zone has a considerable fish abundance (Chasqui & González, 2019).
Sample collection and analysis
We chose five sites from the monitoring stations previously established by the PNNCPR with depths between 35 and 70 m covering most of the Bajo Fríjol area (Figure 1). Diurnal samplings were made in August (E1, E5, and E7) and December (E2 and E4) 2016 according to the PNNCPR monitoring schedule. We took three samples from each site (N=15) using a device designed to collect the seawater right on top of the mesophotic reef substrate (Contreras-Vega, 2017; Contreras-Vega, et al., 2020). We filtered 24 L of seawater with a mesh size of 45 urn (Figure 2) and deposited the 250 mL extract in plastic bottles preserved in 4% borax-neutralized formalin.
Each sample was sedimented for 72 hours. A 50 mL extract was used for the observation and taxonomic identification of zooplankton. The individual count was made using an optic microscope with 10x magnification and the whole sample was analyzed by aliquots in a Bogorov plate.
Data analysis
We calculated the total density (ind/m3), the mean density per station, the percentage of abundance, and species richness. For the taxonomic identification of zooplankton, we used the following taxonomic guides: Boltovskoy (1981); Fernandes (2004); Johnson & Allen (2005); Figueroa, et al. (2005); Costa (2006), and Riviera (2006), and the online databases World Register of Marine Species and Radiolaria.org, endorsed at international level.
We inferred the trophic relations between zooplanktonic components based on the information reported in the literature regarding their eating habits; then, we categorized each species according to its trophic group (carnivore, herbivore, omnivore, and non-consumer) and schematized using the graphic editor program Adobe® Illustrator CC 2018.
Results
Community composition and density
We identified 123 taxa (114 species, nine larvae) grouped in 19 zooplanktonic groups: Amphipoda, Appendicularia, Bryozoa, Cnidarian larvae, Copepoda, Doliolida, Echino-dermata larvae, Foraminifera, Hydrozoa, Nauplii, Ostracoda, Polychaeta, Protozoea, Pteropoda, Radiolaria, Rotifera, Siphonophora, Tintinida, and Veliger (Table 1).
Tintinnids registered the greatest richness with 33 species followed by radiolarians with 32. Copepods and foraminiferans had 24 and 14 species, respectively, and 11 groups had just one representative species (Table 2).
Table 2 Total richness, density and percentage of abundance per zooplanktonic group (S.E= Standard error). N=15
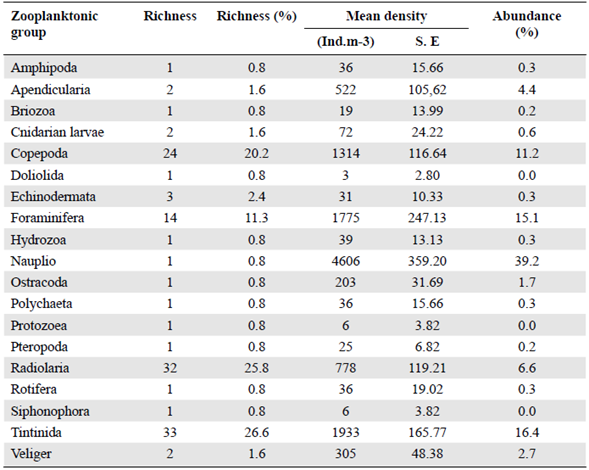
As to the density, we estimated an average of 11728 ind.m-3. Nauplii showed the largest abundance with 39.2% (4606 ind.m-3±359.20) followed by tintinnids with 16.5% (1933 ind.m-3±166,77) (Table 2); in the latter Salpingella sp stood out with 358 ind.m3 (± 62,29) (Figure 3), followed by Eutintinnus lususundae and Eutintinnus medius with 303 and 278 ind.m-3 (±59,83 and 40,62), respectively. Foraminiferans also registered a significant abundance, with 15.1 % (1775 ind.m-3 ±247.13) (Table 2), mostly represented by Globigerina bulloides (678 ind.m-3 ±79,52), the second most abundant species found in this study (Figure 3). Copepods' abundance was 11.2% (1314 ind.m-3±116.64), represented especially by Cyclopoid and Poecilostomatoid copepods. The most abundant species from these orders were Oithona spp. (322 ind.m-3±71.39) and Oncaea spp. (255 ind.m-3 ±60.11), respectively.
Composition and abundance per sampling site
The highest richness was registered on E1 with 85 taxa. Radiolarians were the richest group in this sampling site with 23 species. E7 had the highest number of copepods (21) while E4 had the most tintinnids with 20 species. E4, E5, and E7 had similar richness (between 72 and 73 species) and the lowest richness was registered on E2 (71 species) (Figure 4).
As to the abundance, again, the highest number of individuals (13,916 ind/m3±419.43) was registered on E1 followed by E5 and E7 with 12,819 and 12,430 ind/m3 (±1503.917 and ±961.14), respectively. E7 had the highest abundance of copepods (1,722 ind/m3±163.85) and foraminiferans (2,930 ind/m3±519.59). Tintinnids were most abundant in E1 (2,652 ind/m3±444.66), as well as radiolarians (1,319 ind/m3±422.31) while Nauplii were most abundant in E5 (6,056 ind/m3±860.94). Nauplii were the most abundant in all the stations, and tintinnids, radiolarians, foraminiferans, and copepods were identified on all of them (Figure 4).
Composition and abundance per trophic group
The community was composed of 56% herbivores (3,283 ind.m-3±225.28), 36% omnivores (2,111 ind.m-3±182.01), 8% carnivores (442 ind.m-3±60.83), and <1% non-feeders (19 ind.m-3±9.00). Among herbivores, nauplii represented 50% of the individuals followed by tintinnids with 21%; carnivores were mostly copepods representing 81 % of the individuals and radiolarians were the most abundant omnivores with 37% (Figure 5).
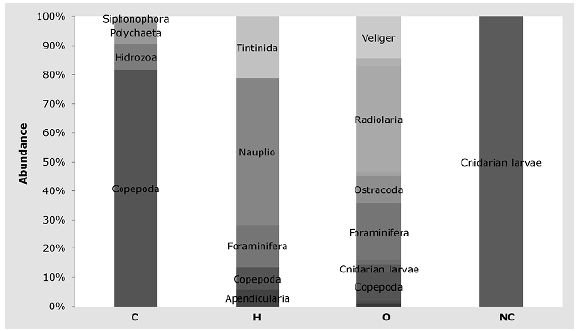
Figure 5 Percentage abundance by trophic group. C: Carnivores, H: Herbivores, O: Omnivores; NC: Non consumers
Regarding species richness, herbivores had 61 taxa: 54% tintinnids and 21% copepods. Omnivores had 50 species of which 81% were radiolarians. Carnivores had nine taxa: 66% of them copepods. Non-feeders were represented only by planula larvae (Figure 6).
Trophic interactions inside the zooplankton community
In this community, the energy flowed from the phytoplankton and bacteria to the zooplankton starting from herbivores (tintinnid, nauplii, some foraminifera, and some copepods), continuing to omnivores (radiolarians, some foraminifera, and some copepods), and then to predators (Polychaeta, cnidarian, some crustaceans, and hydrozoan). The energy finally went to corals and other zooplanktivores (Figure 7).
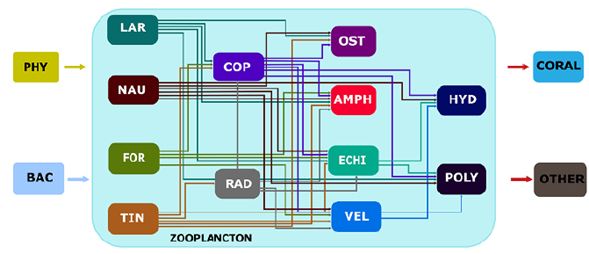
Figure 7 Conceptual model of the trophic interactions within the zooplankton community. PHY=Phytoplankton; BAC= Bacteria; LAR= Larvacea; NAU= Nauplii; FOR= Foraminifera; TIN= Tintinnida; COP= Copepoda; RAD= Radiolaria; OST= Ostracoda; AMPH= Amphipoda; ECHI= Echinodermata larvae; VEL= Molluscan veliger; HYD= Hydrozoa; POLY= Polychaeta larvae
Discussion
Tintinnids had the greatest richness (33 species), as their distribution is cosmopolitan and they are found until 100-m depths (Boltovskoy, 1981; Pierce & Turner, 1993; Thompson, et al., 1999; Thompson, 2001), and radiolarians followed with 32 species; these species show greater diversity in tropical zones with high richness and low abundance, although the latter usually grow in depth (Anderson, 1994; Armstrong & Brasier, 2005).
The large number of nauplii found indicates the continuous reproductive cycles typical in tropical species (Boltovskoy, 1981). These organisms can exceed copepodites and some adult copepods in number, tripling its abundance in some cases (Lang & Ackenhusen-Johns, 1981; Webber & Roff, 1995; Thompson, 2001; Thompson, et al., 2013), just as observed in Bajo Fríjol.
We found a large number of tintinnids in the community, which confirms the assertion by Boltovskoy (1981), Pierce & Turner (1993), Thompson, et al. (1999), and Thompson (2001) that they are one of the main microzooplankton groups with a cosmopolitan distribution and an important role in the secondary production taking place in all seas since it is estimated that they consume up to 67% of the carbon produced by phytoplankton and they can regenerate 10 times more phosphate than copepods due to their high metabolic rate and renew nutrients at high rates.
On the other hand, Foraminifera greatly prefer clean waters with no suspended organic matter and salinity levels between 32 and 42 ppm. Besides, they can reach their maximum size when temperature, salinity, and other ecological parameters are optimal (Boltovskoy, 1981), which makes them excellent bioindicators of water quality (Hallock, et al., 2003).
There are no reports on planktonic radiolarians and tintinnids from Colombian Caribbean oceanic zones, mostly because research efforts have focused on the study of mesozooplankton and the net size used for these (>100μm) does not allow the retention of radiolarians and tintinnids (Thompson, 2001; Vanegas & Arregocés, 2015), which also applies to nauplii, as nets of bigger mesh sizes than those used in our study would not allow the retention of early-stage individuals (Thompson, 2001).
Although some authors have suggested that nauplii could become omnivores if resources are available, most of them use phytoplankton (dinoflagellates and diatoms) as their primary feeding source (Anderson, 1994; Blachwiak-Samolyk, et al., 2007). Most of the herbivore species were tintinnids, which was expected because their grazing on phytoplankton can often excel other bigger consumers (Capriulo & Carpenter, 1983; Thompson, 2001). Their trophic spectra include bacteria, flagellates, coccolithophores, and diatoms (Boltovskoy, 1981; Capriulo & Carpenter, 1983; Thompson, 2001). The dominance of herbivores observed could be related to certain physical and chemical events such as the deep chlorophyll maximum reported for the Colombian Caribbean about 35 m from the sampled area (Gutiérrez, 2010; Navas, et al., 2010).
Radiolarians had the highest number of omnivores. This group has a broad trophic spectrum, including bacteria, detritus, protozoans, and some crustacean fragments (Boltovskoy, 1981; Armstrong & Brasier, 2005). As for carnivores, they were mostly represented by copepods, mainly Cyclopoida (Oithona) and Poecilostomatoida (Oncaea, Farranula and Corycaeus) (Boltovskoy, 1981; López, 2008; Gutiérrez, 2010).
The representation of trophic interactions was based on the trophic spectra of the main zooplanktonic groups found in the study. Tintinnids, larvacea, nauplii, and foraminiferans were located in the first place because they feed mostly on phytoplankton and bacteria (Boltovskoy, 1981; Capriulo & Carpenter, 1983; Schiebel, et al., 1997; Thompson, 2001; Wilson & Hayek, 2019). Radiolarians followed in the second level because, even when they feed mostly from bacteria and phytoplankton, depending on the resource availability, they can be considered omnivores consuming other heterotrophs (Boltovskoy, 1981; Anderson, 1994; Capriulo & Carpenter, 1983). Copepods were placed at the same level because they included representatives from all trophic groups (Boltovskoy, 1981; López, 2008). Ostracod, amphipods, and echinoderm and veliger larvae were located in the third place because they included omnivore representatives (Boltovskoy, 1981; Gutiérrez, 2010). Hydrozoans and polychaetes were located at the higher level as they are mainly carnivores (Boltovskoy, 1981, Blachowiak-Samoly, et al., 2007; Gutiérrez, 2010).
The energy transferred from one zooplanktonic group to another finally reaches the coral polyps. Zooplankton becomes the principal feeding source for most organisms in meso-photic environments, such as benthic organisms (octocorals, sponges, and echinoderms, among others) and demersal zooplanktivores like fishes (Lesser, et al., 2009; Kahng, et al., 2010; Bejarano, et al., 2014; Bessell-Browne, et al., 2014; Nir, et al., 2014). Considering the importance of corals' structuring feature, zooplankton plays a key role in maintaining the reef three-dimensionality and, therefore, the species associated with these ecosystems.
Conclusions
Crustaceans (especially nauplii and copepods), tintinnids, and foraminiferans were the most abundant organisms while tintinnids and radiolarians had the greatest species richness. Herbivores dominated both in composition and abundance with 50 and 78%, respectively. The conceptual model we built suggests that the energy flows from the phyto-plankton and bacteria to the zooplankton starting in herbivores (tintinnids, nauplii, some foraminiferans, some copepods), continuing to omnivores (radiolarian, some foramini-ferans, some copepods), then to predators (polychaetes, cnidarian, some crustaceans, and hydrozoans), finally reaching the corals and other zooplanktivores.
This article offers the first insights regarding the zooplankton associated with MCEs in the Colombian Caribbean, as well as a world list of zooplankton species associated with MCEs, and the first reports of planktonic radiolarians and tintinnids from oceanic zones and MCEs in the Colombian Caribbean.