Introduction
In Colombia, lentic systems such as lakes, lagoons, marshes, swamps, and reservoirs represent ~2.5 % (2,825,800 hectares) of the continental territory, of which 62,200 hectares correspond to reservoirs (IDEAM, 2019). Reservoirs, that are artificial and formed by river damming or accumulation of water in a valley using a dam, cover only 305.7 million hectares worldwide (Deemer, et al., 2016). Nevertheless, due to their capacity to store water, these reservoirs supply a high demand for water resources for human consumption, agriculture, and livestock (Lindström, et al., 2012). Also, many of them provide electromotive energy and are subject to economic activities such as tourism, aquaculture, and artisanal fisheries, among other ecosystem services (Tortajada, 2015).
Unlike temperate latitudes, seasonal changes in highland tropical ecosystems are more sensitive to the variability of the hydrological cycle than thermal contrasts (Catalan & Donato, 2016). For example, meteorological components such as wind speed, solar radiation, and cloudiness, among others, may exert a significant influence on the physicochemical properties of the water column to which plankton communities subsequently respond (Duque & Donato, 1992). A simple and traditional way of researching some limnological aspects has been through the recording of environmental variables coupled with an analysis of the key biological communities (Verniest & Greulich, 2019). In this sense, phytoplankton has been, perhaps, the best-studied hydrobiological component due mainly to its rapid physiological response to the environmental conditions, as well as to its ecological role in the production of molecular oxygen and the transference of organic matter to higher trophic levels (Pinilla, et al., 2000 and references therein). Also, phytoplankton is relatively easy to sample in the field and analyze in the laboratory (Ramírez, 2000).
Although biogeographic barriers between tropical biomes favor a phytoplankton structure with endemic and cosmopolitan species, some populations also occur in certain areas or periods that reveal a particular environmental origin or condition (Coesel, 1983). Therefore, the combined study of physicochemical dynamics and phytoplankton structure contributes to the validation of existing information on ecological succession patterns and those bio-indicator species, independently or sorted into functional groups (Pulido-López & Pinilla-Agudelo, 2017; Hernández, et al., 2020). The use of functional group assignments not only contributes to the understanding of how algae are distributed, but also provides ecological information by considering grouping by habitat preference, trophic specialization, and eco-physiological properties, among others (Reynolds, et al., 2002). Given that there are very few published studies in Colombia rendering data on the continuous monitoring of these limnological properties and even less of the functional characterization of algae, the knowledge of ecological interactions and the current trophic status of aquatic ecosystems remains poorly understood. For this reason, sporadic efforts that investigate temporal fluctuations at a monthly or seasonal scale contribute valuable information.
This study was conducted in El Neusa reservoir, a Neotropical highland dam located in the Colombian Andean mountains. This aquatic system is one of the three principal reservoirs, along with Tominé and Sisga, which feeds the water distribution system to the northern zone of the Bogotá Plateau (Jiménez-Aldana & Santana-López, 2017). The amount of published limnological information in El Neusa reservoir has been limited so far, as well as efforts for assessing its current trophic state and understand biological responses in the face of environmental variability. The purpose of this study was to investigate the monthly dynamics (from July to October 2004) of the phytoplankton community both at taxonomic and functional group (FG) composition levels, and its relationship with some environmental variables. Our hypothesis was based on the expectation that the phytoplankton FGs composition is a consequence of the hydrological regime and water column stratification conditions. We also examined the dominant FGs to contrast the previously elucidated trophic state in this reservoir.
Materials and methods
Study area
El Neusa (maximum depth 38 m, average depth 6 m, volume 118 millions of m3, surface area 9.6 km2, and catchment area 135 km2) is an artificial reservoir situated at 2,977 m altitude and located at 5° 08' 30" N and 73° 58' 28" W in the jurisdiction of the municipalities of Cogua and Tausa in the department of Cundinamarca, Colombia (Márquez & Guillot, 2001). It was built in 1952 by damming the rivers Las Juntas, Cubillos, and Siguatoque, which are currently their main tributaries. According to the climatology and the hydrological cycle (from 1953 to 2003), this region presents a bimodal regime of precipitation with two relatively drought seasons (monthly multi-annual average rainfall <80 mm) lasting from December to February and July to September, and two rainy seasons (monthly multi-annual average rainfall >90 mm) from April to June and October to November (Corporación Autónoma Regional de Cundinamarca-CAR, 2005). Near the littoral, some agricultural subsistence crops and touristic activities prevail while inside the reservoir, trout farming is carried out, as well as some sport fishing and other recreational activities (García & Matallana, 2016). The littoral and shallow areas of the reservoir are usually covered by a dense amount of non-native macrophyte, Egeria densa, which is eventually removed when its biomass becomes excessive (Carrillo, et al., 2006).
Sampling and lab analysis
The sampling was carried out monthly from July to October 2004. According to the meteorological station Lagunitas (No. 21201190), located at 10.8 km from the study area, values of monthly cumulative precipitation were lowest (33 and 45 mm) in July and August 2004 and highest (72 and 89 mm) in September and October; although the second maximum peak of rainfall was delayed until November (116 mm) (IDEAM, 2017a).
We studied four sites inside the reservoir (Figure 1): two corresponded to shallow littoral zones and were designated as Las Juntas (5° 11' 02'' N, 73° 55' 47'' W) and Cubillos (5° 09' 04'' N, 73° 58' 47'' W) given their proximity to the mouth of those two rivers; the remaining sampling sites were located in two areas comprising both zones, limnetic and hypolimnion, in the central and southern parts of the reservoir, which are locally named as Chapinero (5° 09' 43'' N, 73° 57' 02'' W) and the Dam (5° 08' 15'' N, 73° 58' 02'' W), respectively.
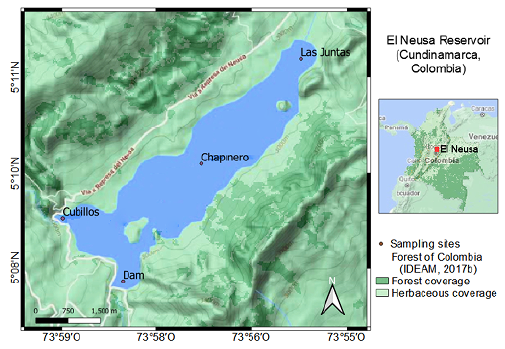
Figure 1 Location map of the study area in the El Neusa reservoir. The sampling sites were Las Juntas, Chapinero, the Dam, and Cubillos surface. The map is a composition of Google Terrain (2020) (Magna Sirgas georeferencing) and forest maps provided on the IDEAM webpage (2017b).
Physicochemical data (temperature, dissolved oxygen, pH, dissolved solids, and suspended solids) were obtained by the use of multiparameter data sonde (Hydrolab Surveyor® 4). We estimated the depth of the mixing layer (MLD) in the epilimnion zone of the Dam based on the criterion of the isothermal layer. The MLD was defined as the depth at which the temperature difference (AT) with respect to the surface value remains less than 0.8 °C (Kara, et al., 2000).
We collected water samples for analysis of nutrients, chlorophyll α, and phytoplankton structure manually at the surface (0.5 m). Additionally, we obtained one set of discrete samples from the water column of Las Juntas (from 1.5 to 2 m depth) and two from Chapinero and the Dam with a horizontal Van Dorn bottle at variable levels depending on the bottom and the euphotic layer (1% surface irradiation -Io-) depths, the latter defined as ~2.7 times the Secchi disk depth. The deepest and intermediate samples from Chapinero and the Dam, respectively, corresponded to the base of the euphotic layer (1% Io) while the deepest sample from the Dam was always immersed in the aphotic zone. For nutrient and chlorophyll α analyses, volumes between 0.5 and 1 L of the samples were filtered through glass fiber filters (Whatman GF/F) and stored at -20oC. The concentrations were obtained by fluorometric and spectrophotometric techniques, respectively (Rice, et al., 2012). The equipment, technical specifications, and analytical procedures to achieve these environmental data are described in Hakspiel-Segura, et al. (2015; 2017), who published most of these data. Total nitrogen (TN) in the dissolved fraction was assessed through the micro-Kjeldahl method plus the sum of nitrite and nitrate while total phosphorus (TP), also in the dissolved fraction, was determined colorimetrically by digestion with nitric acid-sulfuric acid and subsequent reaction with ascorbic acid (Rice, et al., 2012). Phytoplankton samples were destined both for qualitative and quantitative analysis.
For taxonomic identification, surface water was concentrated through a 30 um net and fixed immediately in Transeau solution. Samples for organism abundance analyses were fixed with Lugol's solution at a 1% final concentration. At the Jorge Tadeo Lozano University's limnology laboratory, we dispensed known volumes of the fixed sample in 10 ml sedimentation (Utermõhl) chambers for at least 12 hours before analyzing them with an inverted Olympus CK2 microscope (Sournia, 1978). Taxa were identified at 100X, 400X, and 1000X total magnification to the lowest possible taxonomic level while organism counting was made at 400X. We used Parra, et al. (1982 a, b, c, 1983 a, b), Komarek & Fott (1983), Krammer & Lange-Bertalot (1986), and Popovsky & Pfiester's (1990) taxonomic keys. To visualize the trends of different types of phytoplankton, they were grouped up to the class taxonomic hierarchy and functional groups (FG), this last considering that the taxa shared morphological, physiological, and phenological features according to Reynolds, et al. (2002) and Padisák, et al. (2009) criteria.
Statistical analysis
We used non-parametric analysis as the environmental and biologic variables were not homoscedastic or normally distributed, even after the data were transformed to log or square root. A non-parametric Friedman's analysis of variance (ANOVA) and Kendall's coefficients of concordance (W) were used to assess the variation in rank abundance of phytoplanktonic groups among multiple repeated measures within sampling sites and months. Kendall's W values of 0 represent no agreements, whereas W = 1 represents full agreement (Kendall & Babington-Smith, 1939; Legendre, 2005). The Spearman's (p) rank correlation coefficient was calculated to establish the association between physicochemical variables and phytoplankton group abundance. We performed a canonical correspondence analysis (CCA) to construct a set of orthogonal latent variables which better explains the variation in the taxa abundance allowing for a significant understanding of environmental conditions in which some representative taxa or FG inhabit successfully (Johnson & Altman, 1999). Dominant phytoplankton species and FGs were selected for the CCA based on their frequency (in more than 78 % of all sampling locations) and average abundance (more than 300 individuals L-1) during the study period. A Monte Carlo permutation test was also performed to examine the significance of the CCA axes (Ter Braak, 1986). Spearman's rank correlation coefficients and Friedman-ANOVA analyses were conducted using STATISTICA v. 8.0 software (StatSoft, 2008) while the CCA for phytoplankton and environment relationship multiparametric analysis was performed using PCORD v6.0 software (McCune & Mefford, 2011).
Results
Environmental background
The meteorological and physicochemical parameters of the study area and water column are summarized in table S1,https://www.raccefyn.co/index.php/raccefyn/article/view/1326/3105 as supplementary material. During the study period, rainfall always exceeded total evaporation levels. However, in September-October, evaporation barely reached 23% to 30% of the accumulated monthly rainfall compared to July (47%) and August (90%). The MLD fluctuated from 5 to 8.5 m in July-August and 4.3 to 4.5 m in September-October. This behavior coincided with a gradual increase in temperature and a slight decrease in pH and dissolved oxygen. The pH was positively and significantly correlated with temperature and negatively with conductivity (Spearman ρ = 0.64 and -0.60, respectively, p<0.05, n = 32). Dissolved oxygen tended to decrease with depth reaching values near hypoxia (<1.9 mg L-1) in deeper waters (20 - 27 m) for most samplings, except in August when a minimum value of 4.0 mg L-1 to 24 m depth was obtained.
No vertical and horizontal trends in inorganic nutrients were detected. Most of the nitrite and nitrate measures from the two first months were below the detection limit, so the ammonia contributed largely to the dissolved inorganic nitrogen (DIN). This condition was the opposite in September-October when the oxidized forms contributed on average with ~60 ±16 % of the DIN. Both nitrate and nitrite showed a positive significant relationship with water temperature (Spearman ρ = 0.44 and 0.35, respectively, p<0.05, n = 32) and with total dissolved solids (DS) (Spearman ρ = 0.50 and 0.54, respectively, p<0.05, n = 32) while ammonia was inversely correlated to water temperature (Pearson r= -0.65, p<0.05). The largest value of nitrate and nitrite were detected in October at the Cubillos River following an increase in the total volume of the reservoir (CAR, 2005). TN maintained relatively high values from July to August, as reflected in the negative significant correlations with temperature, nitrate, and nitrite (Spearman ρ = -0.35, -0.41 and -0.50, respectively, p<0.05, n = 32). TP was positively associated with TN and dissolved inorganic phosphorus (DIP) (Spearman ρ = 0.36 and 0.42, p<0.05, n = 32), showing relatively wide fluctuation among sites and months, except in October when TP and TN showed consistent lower values in all locations. The concentrations of phosphorus fractions were not entirely congruent with each other explained by the conceptual fact that, eventually, there was 41 % of samples showing lesser content of phosphorus in the TP than the DIP. This feature could be related to limitations for the release of scavenged phosphorus from organic matter during the digestions of recalcitrant material. Overall, DS was lower in August than in the remaining sampling months. Particularly, the total suspended solids (SS) showed the lowest monthly average value in July, between 3 and 17-fold lower than the remaining monthly average concentrations. Both DS and SS were weakly but significantly correlated with each other (Spearman ρ = 0.36, p<0.05, n = 32).
Phytoplankton community
The phytoplankton community in this reservoir was represented by 111 species belonging to nine classes (Table S2,https://www.raccefyn.co/index.php/raccefyn/article/view/1326/3106). These groups corresponded to Chlorophyceae (36 species), Euglenophyceae (13 species), Bacillariophyceae (14 species), Conjugatophyceae (25 species), Cyanophyceae (11 species), Chrysophyceae (three species), Dinophyceae (four species), Cryptophyceae (three species), and Xanthophyceae (two species), the first four being the richest in species and the highest in abundance.
In general, the abundances of phytoplankton taxa didn't show a significant variation among sites in each sampling month (Kendall's concordance coefficient W = 0.06 to 0.24; Friedman-ANOVA X2 = 4.1 to 17.6, n = 9, df = 7 to 8, p>0.01). The temporal variability of phytoplankton taxa presented a relatively similar trend in most sampling locations as we found no significant differences in their abundance across months (Kendall's concordance coefficient W = 0.07 to 0.4; Friedman-ANOVA X2 = 1.9 to 11.15, n = 9, df = 3, p>0.01) except in Las Juntas (Kendall's concordance coefficient W = ~0.5; Friedman-ANOVA, p<0.01), which fluctuated widely in both abundance and contribution of most phytoplankton taxa.
Chlorophyceae was recorded as the most dominant group in El Neusa with an average contribution of ~72 % to the total phytoplankton population followed by Euglenophyceae, Bacillariophyceae, and Conjugatophyceae with 10.1 %, 6.3 %, and 5.0 %, respectively. Maximum abundance of phytoplankton was recorded in August (average: 8.5 x104 ±2.7 x104 ind. L-1) and September (average: 8.9 x104 ±4.6 x104 ind. L-1) with the highest contribution of Chlorophyceae, Euglenophyceae, and Conjugatophyceae. Euglenophyceae showed its highest abundances in October and, interestingly, Bacillariophyceae abundance diminished substantially in September while a little increase of Conjugatophyceae was detected (Figure 2 a,b).
The most abundant phytoplankton species during the study period were Chlorella sp. (average: 2.4 x104 ±2.0 x104 ind. L-1) and Chloromonas grovei (average: 2.5 x104 ±4.4 x103 ind. L-1), both from the class Chlorophyceae. These species contributed between 54% and 78% of the average monthly abundance of phytoplankton. Generally, Chlorella sp. always maintained high abundances in most samples during the study period. C. grovei abundances were spatially and temporally more fluctuating as it was absent in July and registered high values in August and September. Two species of Euglenophyceae (Trachelomonas planctonica and T. varians) were also among the most frequent species, as well as the diatom Cyclotella operculata and the desmid Cosmarium contractum. Other species such as Nephrocytium agardhianum, Botryococcus braunii, Chlamydocapsa ampla, Sphaerocystis schroeteri, Rhodomonas lacustris var. nannoplanctica, Scenedesmus obtusus, S. acunae, and Aulacoseira granulata were also highly frequent (88% to 100% of the samples), but their monthly average abundance was rarely as high as 2.2 x103 ind. L-1.
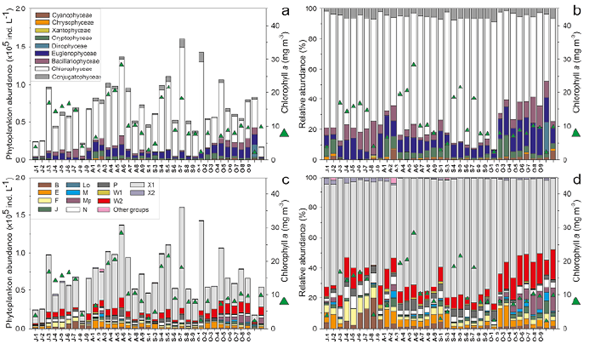
Figure 2 Temporal and spatial variability of the abundance of phytoplanktonic classes and functional groups (FGs) in the El Neusa reservoir between July and October 2004. a. Ind. L-1 of algae classes; b. Relative abundance of algae classes; c. Ind. L-1 of FGs; d. The relative contribution of FGs. Triangles represent the concentration of chlorophyll-α. At axis X: J: July; A: August; S: September; O: October; numbers 1 to 2: Las Juntas from the surface to midwater layer; 3 to 5 and 6 to 8: Chapinero and the Dam, respectively, from the surface to deep layer; 9: Cubillos surface
Cyclotella operculata contributed remarkably within the diatoms group, although it showed a progressive decrease in abundance from July to October. In August, this species dominated this group in most locations together with the central diatoms A. granulata and Cyclotella planktonica. All diatoms species declined abruptly in September and subsequently emerged with a monospecific bloom of Fragilaria capucina (3.2 x103 ±3.1 x103 ind. L-1) in October which accounted for 60% to 94% of the total abundance of diatoms. Eventually, some species of Cryptophyceae (Cryptomonas ovata, Plagioselmis lacustris, and R. lacustris var. nannoplanctica) became abundant in August and October. In September, Closterium aciculare, from the Conjugatophyceae, had a higher abundance; similarly, some species of Chlorophyceae such as B. braunii, C. ampla, Elakatothrix gelatinosa, and Oocystis natans, reached a relatively high abundance in August in all sites.
Phytoplankton functional groups
The spatial and temporal dynamics of the abundance and relative contribution of FGs are shown in Figure 2 c ,d. The microalgae observed in the El Neusa reservoir were grouped into 20 FGs as follows: B, E, F, J, L0, M, MP, N, P, W1, W2, X1, X2, D, G, H1, K, Lm, T, and Y. The last seven groups were generally the lowest in abundance and actually absent in more than 60% of the samples. Members of the W2 and X1 groups were the most abundant in all samples with an average of 10% and 68%, respectively, of the total phytoplankton abundance. Group X1 included three species, two of which were the most dominant in this study, while the W2 group was composed of seven species of the genus Trachelomonas. On average, FGs E, F, and N accounted for about 4% of the total phytoplankton abundance representing the third-largest relative contribution. The groups with more than nine species (F, J, MP, N, and P) dropped into the low-to-moderate range of abundance (range of monthly average: x101 to 5.0 x103 ind. L-1) contributing as a whole only 16% of the average phytoplankton abundance. Group N was the richest in taxa with 16 species followed by group P with 13 species. Both assemblages consisted mainly of Desmidiaceae species but also involved some diatoms. D, K, Lm, T, and Y were monospecific groups with relatively very low abundances (< 1.2 x102 ind. L-1). The rest of the groups had between two and eight species and among them only groups B and E showed moderate abundance (range of monthly average: 5.0 x102 to 4.6 x103 ind. L-1), i.e., over 80% in the samplings. Groups B, F, and N increased their abundances and relative contributions in July while the most important increases of MP and W2 groups were recorded in October and for X1 and P in August and September. Remarkably, the largest abundance averages and relative contributions for groups E and J were observed in August and October, although the maximum values of E were generally associated with Las Juntas.
Spearman's rank correlation coefficients between environment and phytoplankton
Spearman's rank correlation coefficients calculated for the physicochemical variables and phytoplankton group abundance are shown in table 1. As an indirect indicator of phytoplankton biomass, Chlorophyll-α had positive significant correlations with the abundance of total phytoplankton and Chlorophyceae (Spearman ρ = 0.38 and 0.46, respectively, p<0.05). Total phytoplankton abundance was strongly and positively correlated to Euglenophyceae, Conjugatophyceae, and Chlorophyceae (Spearman ρ = 0.65, 0.77, and 0.92, respectively, p<0.05, n = 32). Bacillariophyceae was negatively associated with conductivity, nitrate, and suspended solids and positively related to pH (Spearman ρ = -0.55, -0.40, -0.37, and 0.41, respectively, p<0.05, n = 32). Cryptophyceae and Chrysophyceae were positively correlated to conductivity (Spearman ρ = -0.56 and 0.42, respectively, p<0.05, n = 32) while a negative association was observed between Cryptophyceae and pH and between Cyanophyceae and TP (Spearman ρ = -0.56 and 0.40, respectively, p<0.05, n = 32).
Table 1 Spearman's rank correlation coefficient (ρ) between physicochemical parameters and phytoplankton group abundance in the El Neusa reservoir (n = 32).
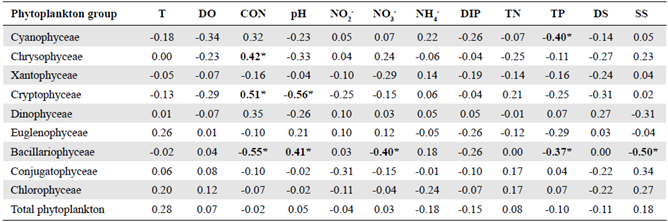
T: water temperature; DO: dissolved oxygen; CON: conductivity; NO2: nitrite; NO3: nitrate; NH4: ammonia; DIP: dissolved inorganic phosphorus; DS: total dissolved solids; SS: total suspended solids; TN: total nitrogen; TP: total phosphorus. Significant cases at confidence interval of 95% are highlighted in bold and marked with asterisks only as a guide.
Concomitantly, we reproduced a nonparametric correlation analysis between the FGs and the environmental variables as shown in table 2. In general, a greater number of significant relationships emerged compared to the environmental matrix (Table S1,https://www.raccefyn.co/index.php/raccefyn/article/view/1326/3105). The Spearman coefficients showed the strongest significant and inverse correlations between conductivity and the groups B and F (Spearman ρ = -0.57 and -0.53, respectively, p<0.05, n = 32) and between pH and the groups E and W1 (Spearman ρ = -0.58 and -0.50, respectively, p<0.05, n = 32).
Table 2 Spearman's rank correlation coefficient (ρ) between physico-chemical parameters and dominant phytoplankton functional groups (FG) in the El Neusa reservoir (n = 32).
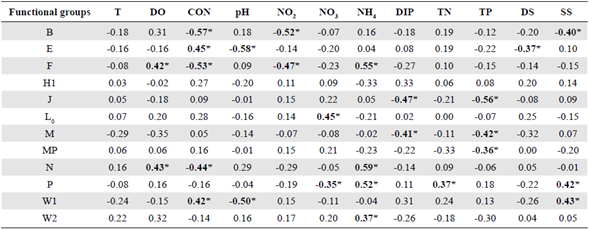
T: water temperature; DO: dissolved oxygen; CON: conductivity; NO2: nitrite; NO3: nitrate; NH4: ammonia; DIP: dissolved inorganic phosphorus; DS: total dissolved solids; SS: total suspended solids; TN: total nitrogen; TP: total phosphorus. Significant cases at confidence interval of 95% are highlighted in bold and marked with asterisks only as a guide. Significant cases at confidence interval of 95% are highlighted in bold and marked with asterisks only as a guide
The strongest positive correlation was observed between ammonia and the groups F, N, and P (Spearman ρ = 0.55, 0.59, and 0.52, respectively, p<0.05, n = 32). Other relatively strong and significant relationships occurred between nitrite and group B and between TP and group J (Spearman ρ = -0.52 and -0.56, respectively, p<0.05, n = 32). There was no relationship between group X1 and environmental factors.
Canonical correspondence analysis (CCA)
The CCA on the 20 most abundant species and 13 physicochemical variables (Table 1) for a set of 32 samples showed correlations that may explain the environmental control on the phytoplanktonic structure at El Neusa (Figure 3). The eigenvalues (λ) on the three axes (λ 1 = 0.18, λ 2 = 0.04, and λ 3 = 0.03, respectively) accounted respectively for 40.0%, 9.2%, and 6.3% of the explained variance. The Monte Carlo permutation test (n = 999 permutations) indicated that these eigenvalues were highly significant (p< 0.01), which validates the use of this method. The first biplot axis was weighted (canonical correlation coefficients: rc) positively by conductivity (rc = 0.61) and negatively by the total suspended solids (rc = -0.57) while the second biplot axis was correlated directly with conductivity (rc = 0.71) and inversely with pH (rc = 0.59). Samplings and environmental variables featured on the CCA ordination biplot (Figure 3 a, c) showed a strong negative correlation between a group conformed mostly by sampling sites during July and the variables of conductivity and total suspended solids for the first biplot axis. Also, a positive correlation was observed between these variables associated with the first axis and the sites that were sampled mainly in September. For the second biplot axis, conductivity was positively associated with all samplings from the surface water at Las Juntas and with some samplings in October; this variable was also inversely correlated with most of the samples collected in Chapinero and the Dam in July and some taken in September. In the same biplot axis, pH had an opposite behavior to that described for conductivity.
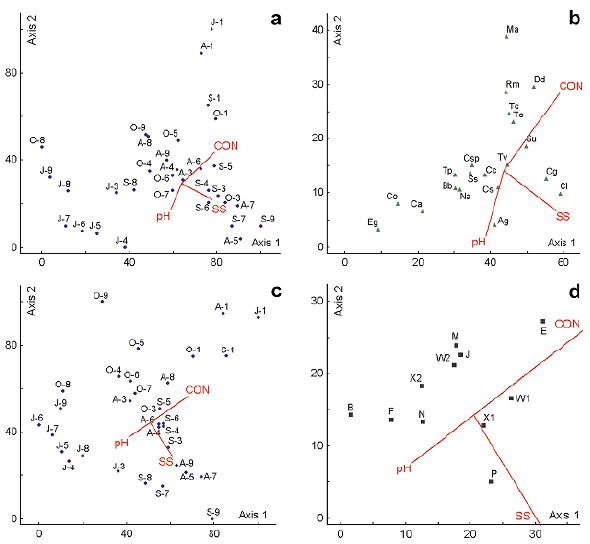
Figure 3 CCA biplots for the two first axis of the relation between abiotic variables (environment) and phytoplankton taxa or functional groups (FGs) in the El Neusa reservoir between July and October 2004. a. Sampling sites of taxa vs. environmental variables; b. Dominant taxa vs. environmental variables; c. FGs sampling sites vs. environmental variables; d. FGs vs. environmental variables. The upper panels refer to dominant phytoplankton species and the lower panels to the dominant FGs. Blue points, green triangles, and black squares stand for the individual samplings (date/site), dominant species, and dominant FGs, respectively; red vectors indicate each of the environmental variables with higher canonical coefficient correlations (rc > 0.3) pointing in the direction of maximum value. Triangles represent the concentration of chlorophyll-α. At axis X: J: July; A: August; S: September; O: October; numbers 1 to 2: Las Juntas from the surface to midwater layer; 3 to 5 and 6 to 8: Chapinero and the Dam, respectively, from the surface to deep layer; 9: Cubillos surface. T: water temperature; DO: dissolved oxygen; CON: conductivity; NO2: nitrite; NO3: nitrate; NH4: ammonia; DIP: dissolved inorganic phosphorus; DS: total dissolved solids; SS: total suspended solids; TN: total nitrogen; TP: total phosphorus. Species acronyms for dominant phytoplankton species: Ag: A. granulata; Bb: B. braunii; Ca: C. ampla; Cs: Chlorella sp.; Cg: C. grovei; Cl: C. aciculare; Cc: C. contractum; Cs: C. raciborskii; Co: C. operculata; Dd: D. divergens; Eg: E. gelatinosa; Ma: M. acaroides; Na: N. agardhianum; Ss: S. schroeteri; Su: S. ulvella; Rm: R. lacustris var. nannoplanctica; Tc: T. caudata; Tp: T. planctonica; Tv: T. varians; To: T. volvocina
The first ordination axis of the CCA (Figure 3 b) showed that taxa such as T. planctonica, C. operculata, C. ampla, B. braunii, E. gelatinosa, and N. agardhianum responded negatively to the increment in conductivity and total suspended solids; on the contrary, Dinobryon divergens, Synura ulvella, C. aciculare, and C. grovei were positively correlated with these variables. At the positive end of the second biplot axis, Mallomonas acaroides, D. divergens, R. lacustris var. nannoplanctica, Trachelomonas caudata, and T. volvocina correlated positively with the increasing conductivity and inversely with the pH and the total suspended solids. For this same second axis, the species A. granulata, C. operculata, C. aciculare, C. ampla, and E. gelatinosa presented an opposite response to that mentioned above.
The CCA ordination performed on physicochemical variables and phytoplankton FGs (Figure 3d) showed that the first canonical axis and the overall test were significant (Monte Carlo test, p<0.03). The eigenvalues of the tree first CCA axes explained, respectively, the 24.0% (λ= 0.046), 15.6% (λ= 0.03) and 4.4% (λ= 0.009) of the total FGs variation among samples. The distribution of environmental variables vectors was similar to that in the CCA shown in figure 3 for the dominant species. In the first biplot axis, group E was positively associated with conductivity and suspended solids while at the other end of this axis the groups B, F, N, and X2 were positively related to pH. In axis two, the groups E, M, J, and W2 were positively related to conductivity and negatively to suspended solids while the P group had the opposite behavior (Figure 3 d). Group X1 was located very close to the centroid of the biplot, which suggests a limited dependence of this group of algae with respect to the reservoir's physiochemical environment.
Discussion
Our results provide a spatial and temporal account of the phytoplanktonic groups and their relationship with environmental variables in El Neusa, a neotropical high mountain lentic ecosystem, during the transition from relative drought (July) to rainy conditions (October), as well as a shift from vertically homogeneous conditions in terms of water temperature, dissolved oxygen, and pH to a more stratified water column (Hakspiel-Segura, et al., 2015). Most of our physical-chemical measurements matched those values and ranges reported for this water body before (Larrahondo-Molina, 1992; Rivera, 1997; Canosa & Pinilla, 1999; Canosa & Pinilla, 2007). Those similarities suggest the absence of pronounced disturbances that could have irreversibly altered the water quality between the 1990s and the 2004-2005 period. However, the 2005 monthly average DIP concentrations showed levels 2 to 5-fold higher than those previously observed, which may have impacted the abundance and composition of the phytoplanktonic community. García & Matallana (2016) reported that for 2015, the monitoring carried out by the CAR revealed that the physicochemical and bacteriological characteristics of this reservoir were within the permissible limits of quality for human use, although some variables such as nutrients may have increased, even within these tolerance ranges.
In our study, the phytoplanktonic composition was temporal and spatially dominated by Chlorophyceae. This group was characterized for being species-rich but with very few abundant ones, which normally prevail under meso-eutrophic conditions (Pinilla, 2000; Peña-Salamanca, et al., 2005). The remarkable numerical predominance of Chlorella sp. and C. grovei are usually associated with high organic pollution and rich nutrient status (Padma-Priya, et al., 2017; Duque & Donato, 1992). The earliest study at El Neusa reservoir performed by Uchima (1987) indicated the predominance of dinoflagellates and euglenoids. Next, Pino (1995) and Rivera (1997) showed that Bacillariophyceae and Conjugatophyceae were the most abundant groups (represented mainly by the genera Aulacoseira, Tabellaria, Staurastrusm, Staurodesmus, and Closterium) associated with the prevalence of relatively low nutrient enrichment, conductivity, and N/P ratios. In a study conducted between February and May 1997, Canosa & Pinilla (2007) confirmed the largest contribution of Bacillariophyceae (~4.0 x104 ind. L-1) and also detected an increasing population of Euglenophyceae (~1.0 x103 ind. L-1), which contrasted with a lower average abundance of Conjugatophyceae, Chlorophyceae, Cyanophyceae, and Dinophyceae (~2.0 x102 to ~7.0 x102 ind. L-1) indicating oligo to mesotrophic conditions. The phytoplankton community with Chlorophyceae as the dominant group confirms that over the years (1988 to 2004) there was a shift towards a higher quantity of nutrients, also supported by the decrease in the relative contribution of Conjugatophyceae over time, which is a sensitive bioindicator group of mostly oligotrophic conditions as reported in several temperate and tropical high-mountain lakes (Coesel, 1983; Banderas-Tarabay, et al., 1991; Rivera, et al., 2005). Besides, different findings on the trophic status at El Neusa reservoir have been registered based on the enumeration of bacterioplankton and virioplankton by epifluorescence microscopy reflecting oligo to mesotrophic conditions (Hakspiel-Segura, et al., 2015; 2017).
In general, the correlation analysis indicated that dominant groups were less sensitive to changes in most environmental variables compared to the less predominant groups. Probably, physicochemical changes among samples were not sufficiently marked so as to cause modifications in dominant groups, therefore, most of these were possibly generalist species that coexist in a wide spectrum of environmental conditions as long as they find minimum nutritional resources. For example, it is known the wide trophic range of Chlorella (Reynolds, 1998). Likely, the dominance and number of individuals per mL of Chlorella sp. may be less evident when its carbon biomass is measured since it is a very small species. A rough estimate of biomass based on a range of carbon values per cell of Chlorella vulgaris under cultivation conditions (Hu, 2014) indicates that the average biomass of this microalga in El Neusa may fluctuate between 550 and 980 pg C L-1. This range is between 1.5% and 2.6% of the bacterial biomass observed during the same months at El Neusa (Hakspiel-Segura, et al., 2015). Compared to mesotrophic Neotropical hydroelectric reservoirs from the Paraná-Paraguay and Paraíba do Sul basins (Silva, et al., 2014), this microalga could contribute up to 5 % of the nano- and micro-phytoplankton biomass.
It was not clear how nutrients controlled the phytoplankton species in the El Neusa reservoir. This apparent decoupling between nutrients and algae taxa could be due to the short time series and the low amount of sampling in our study. However, we do not exclude that this relationship may be obscured by other potential mechanisms of algal abundance control, such as zooplankton grazing and viral lysis, whose impact and seasonal variability are unknown at El Neusa. Besides, along the whole study period, DIN and DIP concentrations were not limiting for phytoplankton growth according to the respective values of 0.08 - 0.1 mg N L-1 and 0.003 - 0.005 μg P L-1 established by Reynolds (1997).
According to the CCA analysis, moderately abundant species, such as E. gelatinosa, C. ampla, and C. operculata, showed their most notable increases during low rainfall months (July and August) under low values of conductivity, suspended solids, and nitrogen. Nutrients were depleted (or under limit detection) as a consequence of limited terrestrial (runoff) or fluvial inputs. The increase in conductivity and suspended solids from August to October along the second axis biplot of the CCA depends directly on the hydrological regime, which affects the runoff of nutrients and other inorganic and organic compounds to the water body from the soil and surrounding terrestrial landscape. For the El Peñol-Guatapé reservoir (Department of Antioquia, Colombia), Gil-Guarín, et al. (2020) also concluded that the hydrological conditions had a determining role in the structuring of phytoplankton communities. The prevalence of pH slightly lower than 7 in this period indicates that the input of allochthonous organic matter favored mineralization processes, which possibly decreased the concentrations of dissolved oxygen (Table 1). These conditions lead to an increase in mixotrophic microalgae populations (Saad, et al., 2016), which is consistent with the responses on the CCA biplot of taxa such as the genera Euglena and Trachelomonas and the species D. divergens and R. lacustris var. nannoplanctica, that can feed on dissolved labile organic matter or planktonic prokaryotes (Yamaguchi, et al., 2012; Ballen-Segura, et al., 2017). Coincidentally, in that period, the abundance of bacterioplankton decreased in most sampling locations while both autotrophic and heterotrophic nanoflagellates, morphologically similar to the potential mixotrophs mentioned above, maintained relatively high densities when measured by epifluorescence microscopy (Hakspiel-Segura, et al., 2015).
The most abundant functional groups (X1 and W2) describe the assemblage of El Neusa matching quite well with the reservoir conditions. The members of FG X1, which was constituted by relatively few species (3 taxa), are nanoplanktonic cells, typical of shallow, well-mixed, meso-eutrophic environments, and tolerant to stratification (Reynolds, et al., 2002). Its scarce relationship with physicochemical variables (no Spearman correlations or association with any parameter in the CCA) is a clear indication that they are organisms with a broad niche, that is, of rather eurioic characteristics. The algae of the W2 group (8 taxa) are bottom-dwelling euglenoids, typically of mesotrophic shallow waters (Reynolds, et al., 2002). The X1 and W2 associations, unlike other functional groups whose organisms respond to primary adaptive life-cycle strategies (C: invasive colonists, S: stress-tolerant, or R: ruderal tolerant to disturbance), may have taxa of intermediate strategies such as CR, CS, or CRS (Moura, et al., 2007). We think that the highest abundances of the invasive motile cells of C. grovei (an X1 member) have probably resulted from a combination of their large capacity of fast-growing and efficient exploitation of available resources (C-strategist), as well as the low removal of its biomass from the water column by active grazing or passive filtration feeding mechanisms (Reynolds, 1997; Elliott, et al., 2001; Reynolds, 2006). Euglenoids of the FG W2, which are principally CS-strategists, might eventually be associated with lower light availability (e.g., hypolimnion or near-bottom waters) given their capacity to exploit rich organic matter environments (Reynolds, et al., 2002; Becker, et al., 2009) for which they dominated throughout the study and increased in October in response to the hydrological behavior and its consequences, as already mentioned.
Due to our study's time scale and the strong predominance of groups X1 and W2, it was difficult to establish a clear phytoplankton successional pattern. However, some less abundant FGs, such as B, F, J, MP, and X2, responded differently to environmental factors in association with the variability of the hydrological balance in the region. On the other hand, the predominance of cyanobacterial groups H1 and S, typical of eutrophic reservoirs or seasonal enrichment conditions in Neotropical systems (Lewis, 1986; Moura, et al., 2007; Rodrigues, et al., 2018), were not observed in El Neusa, which may be partially a result of the role of low temperatures limiting the growth of cyanobacteria rather than the positive effects of a high DIP and the long residence time (~800 days) of the reservoir (Roldán & Ramírez, 2008; Mowe, et al., 2014).
Conclusions
Our results indicate a complex relationship between the phytoplankton group structure and the water body environment. Among the environmental variables, mainly conductivity, suspended solids, and pH varied according to hydrological conditions driving the dynamics of relatively fewer dominant species while the presence of low to moderate DIN and DIP concentrations point to a prevalence of oligo to mesotrophic characteristics while the phytoplanktonic structure dominated by groups X1 and W2 suggests that the El Neusa reservoir may be effectively changing its trophic status. It is expected that nutrient loading increases progressively as a result of natural eutrophication plus the effect of potential anthropogenic forcing (e.g., intensification of subsistence land farming, semi-intensive trout farming, wastewater discharges, etc.). Possibly, the location in a high Neotropical mountain of El Neusa with the consequent lower water temperature slows down the eutrophication process, but in the long term, its water quality is supposed to deteriorate if internal and external factors increasing nutrients are maintained, as has probably been the case in recent years. Our study confirmed that a functional approach helps to understand the processes involved in the functioning and organization of aquatic ecosystems. Thus, the efforts to maintain a monitoring of physicochemical and phytoplankton FGs in water bodies like El Neusa would help to provide a timely diagnosis for decision-making, prevention, and damage restoration in this ecosystem. It would also be advisable to further widen research efforts with a better temporal monitoring resolution and longer study duration.