INTRODUCTION
Ruminants play an important role in food security in developing countries because they can convert fibrous foods such as grass and agro-industrial residues into products of high biological value like meat and milk (Guyader et al., 2016). In these regions, most of the sheep farms present a low level of adoption of technologies, limitations of commercialization, and extensive grazing in pastures with low protein content and high fiber, thus leading to low body weight gain and low income (Vega-Pérez et al., 2017). To solve this, the major trend in ruminant production has been the increase of grain feeding (Dumont et al., 2018). However, these feed sources have disadvantages for small farmers, such as the high price, fluctuations in international markets, and the demand for cereals for biodiesel production (Thompson et al., 2019). Hence, many studies have proved that using tropical trees and shrub leaves in ruminants diets can improve performance and decrease methane production (Vandermeulen et al., 2018). Latin American Tropical dry forests present a high degree of diversity and endemism (Banda et al., 2016). Many of these plants can be used for feeding because they have protein levels greater than 15 % and fiber levels less than 60 % (Pérez et al., 2013). Previous research demonstrated that Samanea saman and Cordia dentata are suitable for ruminant feeding. Since they have high protein levels and lower fiber values (Delgado et al., 2014) than Angleton grass (Dichanthium spp.), a typical grass from the tropical dry forest, characterized for its low protein, high fiber, and low digestibility (Mantilla et al., 2010).
However, there is a lack of information about the effect of including S. saman and C. dentata leaves on intake, digestibility, ruminal kinetics, and nutritional value in ruminant diets. Thus, the aim of this research was to evaluate the effect of partial replacement of Angleton (Dichanthium spp) hay by S. saman and C. dentata leaves on feed intake, in vivo and in vitro digestibility, ruminal kinetics, and blood metabolites in hair lambs.
METHOD
Localization
This experiment was conducted from June to September 2017 at the experimental farm “Las Brisas” of the University of Tolima, in the city of Ibague, department of Tolima, Colombia, located at 4°25'38.4" N + 75°13'40.8" W, 1285 masl with annual precipitation between 1.500 and 2.000 mm and an average temperature of 23,1 °C.
Experimental diets and treatments
The Dichanthium spp was collected at the “Centro Universitario Regional del Norte” of the University of Tolima, Armero, Tolima, Colombia. The hay was chopped into one to three cm of size for ease of feeding. TheS. samanandC. dentataleaves were collected at intervals of 45 days at “Centro de Investigación NATAIMA”,El Espinal, Colombia.
The experimental diets consisted ofDichanthium spp hay with partial replacement byS. samanandC. dentataleaves (Table 1).
T2: 80 %Dichanthium spp hay, 20 % S. saman;
T3: 80 %Dichanthium spp, 20 %C. dentata;
T4: 80 %Dichanthium spp, 10 %S. saman, 10 %C. dentata
Animals and experimental design
This study was carried out following the guidelines of the University of Tolima (agreement of the academic council number 0171 of October 29, 2008) and act number 02 of 2017 of the committee of bioethics.
Twelve castrated Colombian hair lambs (20,15 ± 1,67 kg and an average age of six months) were randomly distributed and allocated to one of four dietary treatments. Each group received one of the diets under 4 x 4 Latin Square experimental design with four treatments, four experimental periods, and three animals for each treatment.
Sample collection
In vivodigestibility was determined by the total collection of feces. Each experimental period was divided into two: 12 days of diet adaptation and five days for data and sample collection. During the adaptation period, the lambs were located in pens for each treatment and fed at 4 % of live body weight. In the collection period, the lambs were housed in metabolic cages equipped with feeders, waterers, and collectors of feces and urine. The diet was adjusted to obtain 5-10 % orts. Feces and orts were collected every morning before feeding and weighed, and samples were taken and frozen for further analysis.
Samples of feeds, orts, and feces were pre-dried for 72 hours in an air circulation stove at 55 °C and ground in Willey type mills (2 mm mesh) and analyzed for Dry Matter (DM), Crude Protein (CP), Ether Extract (EE), (AOAC, 2009); Neutral Detergent Fiber (NDF), Acid Detergent Fiber (ADF), and lignin content (Van Soest et al., 1991), Total Carbohydrates (TC). Non-Fiber Carbohydrates (NFC) were calculated as follows (Equation 1 ) (Sniffen et al., 1992):
Blood samples were collected per animal on the last day of each experimental period. They were analyzed for Plasma glucose, NEFA, and BHBA concentrations using commercial Biosystems® (glucose and BUN) and Randox® (β-hydroxybutyrate) kits and Biosystems A15® automatic blood chemistry system at the Laboratory of Veterinary Diagnostics at the University of Tolima-LADIVE.
Intake and apparent digestibility evaluations
DM, OM, CP, NDF, ADF, EE, TC, NFC, intake, and TDN of each treatment were calculated by the difference between the total of each nutrient contained in the feed offered to the lambs and the amount in the orts, expressed in grams per animal per day (g d-1), Apparent digestibility (AD) of DM, OM, CP, NDF, EE, TC, and NFC was obtained using the equation as follows (Equation 2). where: Nf = nutrient in feed (g); No = nutrient in orts (g); and Nfc = nutrient in feces (g).
TDN was calculated as follows (Equation 3) (Weiss, 1993), where subscript d means digestible.
The DE was estimated at 4,4 Mcal/kg of TDN, and the DE was converted to ME at an 82 % efficiency rate (National Research Council-NRC, 2007).
In vitro digestibility and ruminal degradability
In vitro DM digestibility (IVDMD) was determined based on the technique proposed by Tilley and Terry (1963), adapted to the DAISY II®-ANKOM® artificial rumen. Ruminal fluid was obtained from a rumen-cannulated Gyrolando steer maintained in a pen ofDichanthium sppgrass with water ad libitum. For each feed sample (pre-ground to 1 mm), 0,5 g were placed into F57 ANKOM® filter bags. Then they were then distributed in four glass containers with buffered solutions A and B and ruminal inoculum. Subsequently, they were introduced into the DAISY II® incubator for 48 hours at a constant temperature of 39 °C. At the end of this period, 40 mL of 6 N HCl and 8 g of pepsin were added (EC 3.4.23.1 Sigma®). The samples were left in an incubator for an additional 24 hours. Subsequently, the bags were dried at 105 °C for eight hours; DIVMS was calculated as the difference between the incubated feed and the residue after incubation.
Degradation kinetics of DM was determined in parallel within in vitro dry matter digestibility using the DAISY II® incubator. The incubation times at 3, 6, 12, 24, 48, and 96 hours were considered. The parameters of in vitro rumen degradation of DM were calculated as follows (Equation 4) (Orskov and McDonald, 1979), where, P is the amount degraded at time t, “a” is the rapidly soluble fraction, “b” is the potentially degradable fraction, “c” is the rate of degradation of fraction b.
Potential degradability (PD) was calculated using the following equation (Equation 5) where a and b represent the soluble and degradable fractions, respectively.
The effective degradability (ED) of the DM was calculated using the following equation (Equation 6) where k is the velocity of the passage of particles in the rumen, estimated for each diet, considering the passage rates of 2, 5, and 8 %/hour, values that can be attributed to low, medium, and high consumption levels, respectively.
Statistical analysis
Nutrients intake, digestibility, and blood metabolites data were statistically using the general linear model procedure of SAS software (Statistical Analysis System, version 9,1). The treatment, period, and animal and their respective interactions as fixed effects. When the analysis of variance indicated the existence of differences between treatments, the respective means were compared using the Tukey test with significance declared at p<0,05. Analysis of variance was carried out for in vitro digestibility, in vitro degradability and degradability kinetic parameters were compared using the Tukey test with significance declared at p<0,05.
RESULTS
Intake
DM, OM, NDF intake were higher in the diets containing tree leaves (p<0,01). Furthermore, NDF intake containing T4 was superior to the other diets (p<0,01). The CP intake was lower in lambs fed with the T1 diet, while the lambs fed diets with T2 and T4 showed higher values (p<0,01) (Table 2). The dry matter intake increased between 45 to 59 % in lambs fed with S. saman and C. dentata. The increase of their intake contributes to improving OM, CP, NDF, EE, TC, NFC, and TDN consumption. These findings corroborate the results of Castañeda-Serrano et al. (2018) who reported a similar intake in lambs fed with 25 % of G. ulmifolia, G. sepium, or T. diversifolia leaves with a basal diet of Dichanthium spp hay and rice bran. In our study, the lowest DM intake was obtained with the 100 % Dichanthium spp diet, which contains 5 to 7 % more fiber than other diets. Less fiber in diets with tree leaves might have resulted in less time in chewing and ruminal filling and a higher ruminal outflow that might have caused a higher intake (Niderkorn et al., 2019). Protein intake is the main factor in daily weight gain. We found protein intake with tree leaves increased between 44 to 59 % in the lambs fed with diets with tree leaves (Costa-Germano et al., 2018). Similarly, Marsetyo et al. (2020) reported that lambs fed basal diets of Mulato grass with supplementation of Centrosema pascuorum, Dolichos lablab, or Clitoria ternatea showed higher nutrients intake compared with the control diet but not showed differences between them. This occurred because lambs fed ad libitum can choose feeds, or parts of them, which are of good nutritional quality (Moyo et al., 2019). Otherwise, the results of this study suggest high acceptability of S. saman and C. dentata by ruminants in accordance with Pérez-Almario et al. (2013) who reported high acceptability of S. saman and C. dentata by dairy cows.
Digestibility
DM, MO, NDF, TC digestibility and, IVDMD were higher for diets with tree leaves than the T1 diet, but they did not show differences between them (p<0,01). CP digestibility was highest for the T2 diet, followed by T3, T4, and T1 diet (p<0,01). EE and NFC digestibility did not show a difference between diets (p>0,05). The TDN values for diets with tree leaves were higher than the T1 diet (p<0,01) (Table 3). The highest digestibility of DM, OM, CP, NDF, and TC for the treatments with tree leaves, is consistent with the results of Sio et al. (2018). They evaluated the digestibility of the diets with three levels of Paraserianthes falcataria leaves (10, 15, and 20 %) in a grass basal diet and found that from 10 % of inclusion, it is possible to improve the digestibility of nutrients due to the rapid growth of the microbial population of the rumen becauseS. samanandC. dentatahave high levels of protein and medium of low levels of NDF
Blood metabolites
Glucose values showed no differences between treatments (p>0,05). βHB showed a similar pattern (p>0,05). Furthermore, the BUN of lambs fed the T2 diet showed higher values (p<0,01). (Table 4). The blood urea nitrogen (BUN) values were lower than those established for sheep (Carlos-Leite et al., 2015). Low levels of this metabolite may be due to deficient protein or energy in the diet (De Oliveira et al., 2016). However, the highest levels of BUN in T2 treatment could indicate a better contribution of nitrogen to microbial protein production. Glucose values are closely correlated with dry matter and energy intake. However, in our trial, we did not observe differences between the treatments even though we observed differences for feed consumption, and the energy values were lower for the T1 diet. Regarding the βHB concentrations, these did not differ between treatments, and their values were below the proposed in sheep (Catunda-Vasconcelos et al., 2013) (5,33 to 6,17 mg/dL). According to these results, it can be inferred that the lambs were not in a negative energy balance.
Ruminal Degradability and degradability kinetics
At 96 h the T2 showed the highest degradability among the other diets. On the other hand, the T3 and T4 diets presented higher values than the T1 diet (Table 5). Regarding effective degradability, the highest values were observed in the T2 diet in the three levels of passage rate. The "a” and ¨fraction in the T3 diet was superior to the other diets. However, its “c” fraction was lower. The potential degradability was higher for the T3 diets (Table 6). The “a” fraction shown in tree leaves diets might be attributed to higher CP levels in S. saman and C. dentata, leading to a high amount of nitrogen available in the rumen used to produce microbial protein, the principal source of proteins for ruminants (De Oliveira et al., 2014). Patra and Yu (2014, 2015) showed that saponins found in S. saman (Anantasook et al., 2015) and nitrates found in C. dentata (Garcia et al., 2009), could inhibit methane production, allowing the growth of cellulolytic bacteria and increases the feed degradability and production of volatile fatty acids. Otherwise, in this study, the T4 diet shows the lower “b” fraction. To the knowledge of the authors, there are no studies that evaluated or explained the in vivo interaction between saponins and nitrates.
Table 5 In vitro degradability by hours of dry matter in diets for hair lambs using Dichanthium spp, S. saman, and C. dentata.
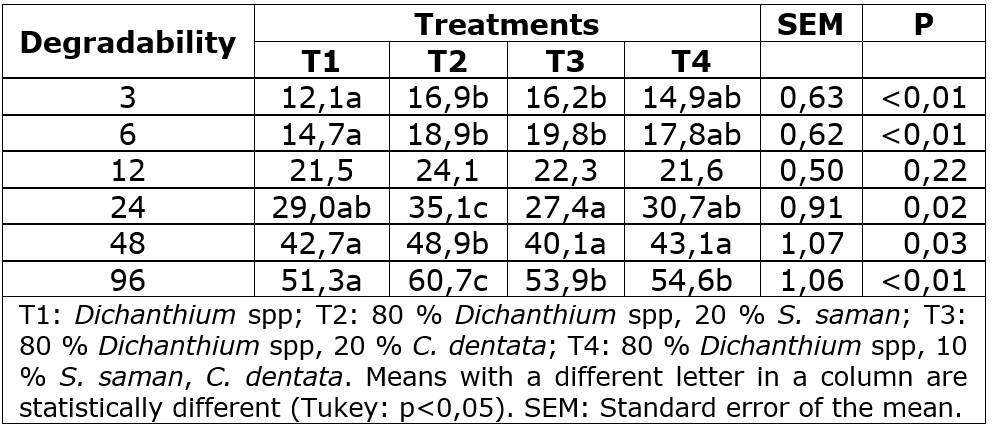
CONCLUSIONS
Intake, digestibility, and degradability of the nutrients improved when S. saman and C. dentata were included in the diet. We demonstrated that their inclusion does not have harmful effects on protein and energy status. Their inclusion is a promising alternative for the supplementation of low-quality grass-fed ruminants in tropical and subtropical countries.
We suggest that this information could be used as basic data for future studies, where conventional protein feeds will be replaced by S. saman and C. dentata.