INTRODUCTION
Quinoa (Chenopodium quinoa Willd.) belongs to the family Amaranthaceae and is mainly grown in the Altiplano and arid areas of Andean region of South America although is currently cultivated on all continents, it also displays wide adaptability to altitudes ranging from 0 to 4000 m, i.e. cold, temperate and warm environments (Garcia et al., 2020). In the case of Colombia, there are two predominant cultivated varieties, Blanca de Jerico and Tunkahuan, which usually have a yield between 1 and 2 t ha-1. Generally, studies on quinoa are focused on genetically improving the seed or cultivars adaptations under different agronomical conditions (Wu et al., 2020).
Quinoa, Chenopodium quinoa Willd, is a pseudocereal that recently, has been under the eye because its high nutritional value, low glycemic index, and its gluten free value (Sun et al., 2021). The use of the grain as a raw material in manufacturing food products, researches are mostly focused on its nutritional, physical and chemical characteristics of the grain. Quinoa protein with great functional properties has been considered a potential source for food fortification or the production of functional foods (Daliri et al., 2021).
Results have revealed that quinoa protein has high amino-acids profile thanks to its balanced blend of lysine, methionine, tryptophane and threonine (Le et al., 2021; Rodríguez et al., 2021) for a better digestibility (Ghumman et al., 2021). Its starch is polymorphic with gelatinization enthalpy between 7,2 J/g and 10,3 J/g; low amylose and highly resistant starch (Roa et al., 2019; Xing et al., 2021). In different quinoa varieties, fats can vary between 3,9 g and 5,2 g (Rodríguez et al., 2021). Additionally, FAO and OMS had declared as a promising cultivar, because its gluten free virtue that could be ingest by many people especially those with coeliac disease (Saavedra and Valdez, 2021). On the other hand, Soy protein beverages are considered safe and can enrich the varied diet of its consumers, as long as, they are considered an additional liquid portion of the diet. They can be ingested by adults and children above two years of age that present with cow’s milk protein allergy or lactose intolerance (Vázquez-Frias et al., 2020). While that, rice protein has higher nutritional quality, true digestibility (TD), and biological value (BV) than soy protein isolate (Han, Chee & Cho, 2015) and other cereals. Since rice protein contains lysine with high-quality amino acid composition, it is hypoallergenic and, therefore, favorable for human consumption (Pakkawat Detchewa et al., 2022).
From a technological point of view, proteins have become a necessary ingredient to be included on new products (Muchekeza et al., 2021). This would be possible thanks to the proteins capacity to make foams and emulsions (Wouters and Delcour, 2019). Consequently, chemical process had been developed to obtain protein isolates and protein concentrates, which are different from each other from its percentage of extracted protein. Preliminary testing allowed to notice that quinoa protein isolate might preserve some of its technical and functional attributes; its efficiency to make emulsions as well as its stability on thermal procedures after extraction. Therefore, the interest in using quinoa protein is not only because its nutritional value, but also on its technical and functionality (Roa et al., 2019).
Some of the strategies to improve functionality and bioactivity of proteins are enzymatic hydrolysis. It consists on, adding specific enzymes to a protein to break peptic chemical bonds to obtain peptides (Deleu et al., 2019). The hydrolysis degree is determined because of reaction conditions like substrate concentration; enzyme/substrate affinity; incubation timing, pH and temperature. Other factor is, the nature of such applied enzyme as in type of enzyme and its specificity. Then, the nature of the used enzyme influences over the hydrolysis degree as well as the resulting peptide (Daliri et al., 2021).
Because of previous explanation, peptides can be classified in: low molecular mass hydrolysates
between 1 and 10 % of DH, and high molecular hydrolysates with DH over 10 % (Villanueva et al., 1999). Low mass hydrolysates are used on food industry as emulsifiers and foaming agents. While high mass hydrolysates are used on specialized food industry because its bioactivity and antioxidant properties (Gómez et al., 2021). This explains why this research worked monitoring the enzymatic hydrolysis from three protein isolates seeds: rice, soy and quinoa. A comparative structure analysis between the native protein and hydrolysates through infrared spectroscopy to determine the conformation changes and connect them with some of the functional properties.
METHOD
This study was made at the Faculty of Agricultural Sciences laboratory at Universidad del Cauca. In Vereda Las Guacas (Popayán-Cauca) 02º45'08.91'' North and 76º55'10.86” West. Altitude of 1.737 m.a.s.l; average temperature of 19 ºC; and annual precipitation of 1.800 mm. Quinoa (Chenopodium quinoa Willd), rice (Oryza sativa), and soy (Glycine max) grains were carefully selected to dispose of contaminants, defective samples, foreign particles and those with phytosanitary damages. Grains were stored on high density propylene bags at room temperature.
Extraction of Protein isolates
Quinoa, rice and soy flours were degrease using ethylic ether as a dissolvent on a Soxhelt system for about 6 hours. Protein isolate extract was the methodology described by Mir et al. (2021) with just a few modifications.
Flour previously degreased were suspended on distilled water 1:10 w/v for 10 minutes. Then, NaOH (2 N) at 9 pH, was added keeping a constant stirring for 30 minutes. Next, sample passed for centrifugation at 8000 rpm for 10 minutes, using a Hermle Z306 (Germany) equipment. Obtained supernatant was adjusted to Isoelectric point at pH 5, using HCl (2 N). Once again centrifugated at 8000 rpm for 10 minutes. Precipitated recovered and stored in freezer at -18 °C for 24hours. To complete process, it was later dried up at 40 °C for 24 hours.
Hydrolysis degree determination
Hydrolysis degree in proteins were calculated following “TNBS instructions” method provided by Biotechnology lab (Galante et al., 2020). After getting a calibration curve with Leucine, hydrolysis measurements were taken. This consists of adding: 128 µL of hydrolysate sample, plus 2 mL buffer at pH 8,2 and 1 ml more of TNBS. Reaction was left on a thermostatized bath at 50 °C for 30 min. Once time passed, reaction is stopped adding 2 mL of Sodium sulfite for 15 minutes. After that, visible-ultraviolet spectroscopy was used to read at a wavelength of 420 nm.
Total hydrolysis followed this procedure: 1 g of protein isolate was put into a test tube to then add 5 mL of hydrochloric acid (6 N). Next, mixture was placed into a sand bath at 110 °C for 24 hours to finally be neutralized with Sodium hydroxide (6 N). Hydrolysis degree was determined by TBNS.
Work schedule consisted in monitoring hydrolysis timing to which a total acid hydrolysis was performed to all three protein isolates. Enzymatic hydrolysis happened using method proposed by Villanueva et al. 81999a) and Villanueva et al. (1999b) with few modifications. To obtain DH greater than 35 %, partial hydrolysis (type 1) consisted in adding 1 g of isolate protein on a 50 mL Falcon test tube. Later, 10 mL at pH 8 buffer was added and mixed on Shaker equipment at 150 rpm y 55 °C. Subsequently, 2,4 L of Alcalase enzyme was pour in and left to react under selected timing for each sample.
Partial hydrolysis (type 2) consisted in neutralizing previous reaction to a pH 7, using (2N) HCl. Afterward, Flavorzyme® was added. Then, reaction was stopped 120 minutes later by heating it up to 85 °C for 15 minutes. Samples were centrifugated; and filtered on 110 mm Whatman paper. Lastly, hydrolysis degree was determined by TNBS (Kristoffersen et al., 2020).
Mid-range infrared spectroscopy
IR spectrum were obtained using IRAFFINITY-1S equipment (Shimadzu Corp.,Kyoto, Japan). Spectrum was performed by reflection mode between 400 cm-1 and 4.000 cm-1 and proportional to over 32 scans with 4 cm-1 and 25 °C temperature. Previous to measurement, each cultivar had a blank background spectrum (Roa et al., 2019). Spectrum analysis was made by using an OriginPro, 7th version. Measurements were fixed and standardized over a base line between 0 and 1 (shown on figures) to display highest transmittance peaks. Deconvolution was performed on spectrum using Fourier Transform to determine protein secondary structure changes (band 1.600 cm-1 to 1.700 cm-1). Later, a modeling of the Gaussian function spectrum was applied (Perczel et al., 1992). Starch concentration was studied from its formation on short range between 875 cm−1 to 1175 cm−1 band (Roa et al., 2020). Lipids present on 2800 cm-1 and 2900 cm-1 range. All samples were analyzed by triplicate.
Statistical analysis
All experiments were conducted in triplicate and results were expressed as means and standard deviations. Data were evaluated by analysis of variance (ANOVA) using SPSS (version 16.0 for Windows). Tukey Test was used for multiple comparison tests at statistical significance of p< 0,05.
RESULTS
Degree of Hydrolysis on Quinoa, Soy and Rice protein isolates
Preliminary testing allowed to determine that hydrolysis degree (DH) to both Enzyme/Substrate [E/S] had a 5 % ratio. Hydrolysis time of 180 minutes was adjusted according to authors like: (Villanueva et al., 1999a; Montoya et al., 2020; Mahdavi et al., 2019; Leni et al., 2020). Alcalase hydrolysis reaction in rice (RPI) up to 60 minutes did not show significant differences: 0,54 % DH (t = 0 min) to 29,1 % DH (t = 60 min). When adding Flavorzyme®, DH rose to 38 % at 80 min. After such time, no differences were relevant to include on DH (Fig 1). Reaction stopped at 180 minutes after. SPI hydrolysis (soy) ran for 180 minutes as well. Meaningful differences found under Alcalase treatment: from 2,4 % DH (t = 0 min) up to 37,1% DH (t = 60 min). Upon adding Flavourzyme, DH reached 40,3 % at 80 minutes and then to 42,5 % when reached 180 minutes (Figure 1).
Quinoa exhibited a different behavior. At the begging of the phase 1, changes happened from 7,40 % DH (t = 0 min) to 33,7 % (t = 60 min). Second phase with a DH of 42,1 % (t = 80 min) and ended in 46,0 %. Values on first stage of hydrolysis process on Quinoa Protein Isolate (QPI) and Rice Protein Isolate (RPI) are slightly lower (DH 34,7 %), to those reported by Villanueva et al. (1999b), although they are higher to the obtained by Xiaoxue et al. (2021).
On the other hand, Soy Protein Isolate (SPI) with a 40,3 % surpasses the values found for the authors. Its conduct on first stage one hydrolysis (0 time) is attributed to the initial extraction technique of protein isolates. In quinoa’s situation, acidic procedures boosted an initial hydrolysis greater on the other two commercial protein isolates. Alcalase’ digestion, managed to rise the number of N-terminal to give room to locations with exo-proteins activity to, therefore, move to second stage of enzymatic treatment.
Increment of the DH on hydrolysates on first stage happened thanks to Flavorzyme. Result of a DH 54,3 % are lower to the one reported by Villanueva et al. (1999). However, degrees of hydrolysis in quinoa and soy are minor to those reported by Hesam et al. (2021) (GH: 26,3 %). It is essential to mention that the final hydrolysis degree is determined by substrate concentration; correlation between enzyme/substrate; incubation time; and physical and chemical conditions like temperature and pH. Once the enzymatic hydrolysis of quinoa, rice and soy is finished at 180 minutes, samples were taken to mid-range spectroscopy equipment to review any changes on their secondary structural organization of protein isolates.
Figure 2 shows spectra of such secondary structures of protein isolates and their hydrolysate Secondary structures allocation was based on previous publish protocols by Yang et al. (2015). (-sheets were identified between 1620 cm-1 to 1640 cm-1. Increase of structural intensity was observed because of hydrolysis process (Figure 2). Hydrolysis makes possible that some structures change and become new structures on (-sheet. It is important to mention that between 1640 cm-1 a 1660 cm-1 rage, random spiral structure intensity was reduced for the quinoa’s protein isolate. It is possible that such structures change into more organized ones.
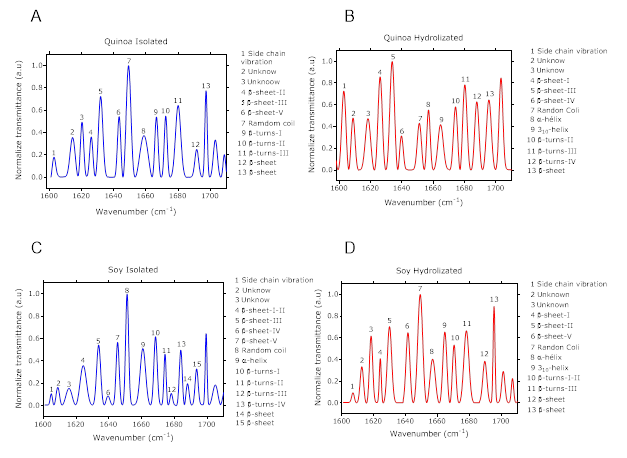
Figure 2 Spectrum of quinoa protein isolate (A), Spectrum of soy quinoa protein hydrolyzed (B), Spectrum of soy protein isolate (C), Spectrum of soy quinoa protein hydrolyzed (D), with their secondary structure.
Figure 2B, details that D structure of 310 helix on both hydrolyze, quinoa and soy, structures not observed on hydrolysates (Figure 2A, C), demonstrating that (-turns structures between 660 cm-1 and 1680 cm-1 were more sensitive to enzymes effects. None specific tendency was identified. Also, some of the functional phenolic compounds may vibrate on this specter range, as the aromatic chemical bond on C - H (curve out of plane) at 1850 cm-1 is characteristic on poly-phenolic compounds. However vibrational frequencies more representatives were between 1563 cm-1, 1570 cm-1 and 1577 cm-1 assigned to the vibration C = CO aromatic groups.
Figure 3 displays a comparative analysis for (-sheet, (-turns, (-helix and (-type to each one of isolates. For soy and rice isolates, a diminishing tendency is observed on -sheet bands during hydrolysis process. However, (-sheet structures behavior in quinoa protein, is different (Figure 3: (-sheet) (Haque et al., 2016). It is interesting to make note that hydrolysis treatment did not affect (-type structure on rice protein (Figure 3:(((type) (Zang et al., 2019).
Analysis over this correlation made evident a substantial link between the structure’s behavior β-turns and (-helix. Hydrolysis process causes and increment on (-turns for soy hydrolysate structures (Klompong et al,. 2007) as well as in quinoa; although, simultaneously, there is a reduction on (-helix structures on same hydrolyzed samples. This might be possible because the structure adjustment of (-helix to (-turns (Pal Singh et al., 2016). On the contrary, the opposite effect was detected on rice protein.
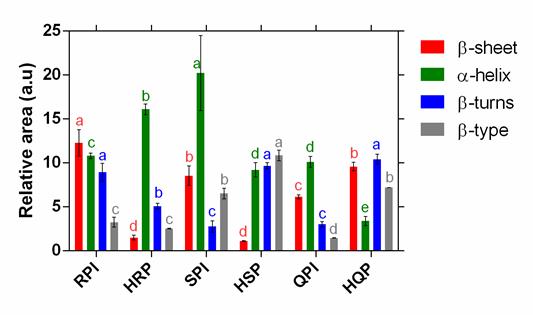
Figure 3 Comparative analysis of each secondary structure (β-sheet III, β-turns III, β-helix and β-type) of the protein isolate and its hydrolyzed, RPI: rice protein isolate, HRP: rice protein hydrolyzed, SPI: soy protein isolate, HSP: soy protein hydrolyzed, QPI: quinoa protein isolate, HQP: : quinoa protein hydrolyzed . The different letters (a,e) represent statistical differences at p <0.05.
CONCLUSIONS
Enzymatic alkaline action had a greater effect over soy protein, while Flavorizime action is greater over rice and quinoa. This could be due to the similarities on protein structure, because rice is a cereal and quinoa a pseudo-cereal. Secondary structures more influenced were (-sheet and (-helix. Finally, it is relevant to mention that agro-industrial potential of this hydrolysate proteins is linked to its functionality and may offer benefits as natural antioxidants; anti-hypertensive; and anti-cholesterolemic properties, as well as improving some products’ texture of broad use in food industry; since hydrolysates expose their hydrophobic or hydrophilic residues, these give them greater solubility and stability at the interfaces.