Introduction
In general, oil and gas traps are not completely sealed, so their accumulation will cause a release of some of the oil and gas to the surface. When oil and gas are clearly visible on the surface, macroseepage traces will give invisible clues of oil and gas traps in the near-surface soil and sedimentary rocks. Historically, the accumulation of seepage is closely related to the presence of oil and gas traps. Therefore, a lot of oil and gas fields in the world have been drilled in leaky areas. In modern exploration projects, macroseeps are often seen as direct clues of source rocks and the formation of convincing evidence of a petroleum system in a given basin.
The use of remote sensing for oil and gas exploration is an extraordinarily complex and systematic undertaking (Panciera et al., 2009; Van der Kwast et al., 2009). A large amount of remote sensing data is required to interpret and analyze the micro-percolation, alteration, and geological basin structures, such as regional structures, strata, and tectonic belts, of oil-bearing areas (Jiang et al., 2011; Wang et al., 2015). Geological, geophysical, and geochemical exploration methods are combined to detect oil- and gas-bearing exploring prospects under the guidance of modern geological petroleum theory (Yi et al., 2016; Cheng and Tong, 2015; Kandpal et al., 2016; Petrovic et al., 2012; Sun, 2012).
Hydrocarbon percolation is a phenomenon in which petroleum and natural gas are accompanied by a dense layer of penetrating rocks (Bateson et al., 2000; Asadzadeh et al., 2016; Cui et al., 2013). The hyperspectral trap area itself is a dynamic balance system (Zhu et al., 2008; Zheng et al., 2015; De Carolis et al., 2014). Hydrocarbon molecules penetrate through the overlying rock stratum in the process of migration under the influence of concentration gradients. Under the effects of pressure gradients, hydrocarbons migrate toward the surface along pathways such as joints, porosities, and cracks (Svejkovsky et al., 2012; Clark et al., 2003; Otremba et al., 2013). Hyperspectral remote sensing exploration technology is based on reflectance spectrometry and oil and gas percolation theory, and is a straightforward approach to detecting oil and gas reservoirs. Since the 1990s, it has been widely used in oil exploration in China and overseas.
Many studies in hyperspectral remote sensing have been conducted, and applied models have been established. The pioneering work in the early 1970s paved the way for the interpretation of remote sensing data using quantum mechanical concepts (Wu et al., 2005). Their work established a link between the reflectance change and the chemical and physical properties of minerals, focusing on its potential use in remote sensing (Hunt et al., 1977; 1979). In 1998, researchers at West Virginia University made use of a mineral alteration anomaly and successfully discovered an oil and gas percolation field (Sabins et al., 1999; Eismann et al., 2007; Heylen et al., 2014). In 1999, utilizing hyperspectral instruments, German researchers identified an anomaly and determined the position of oil reservoirs from the spectral radiation characteristics of high signal-to-noise (SNR) HyMap pixels (Goetz and Rowan, 1981; Gregory and Moore, 1975; Sabins et al., 1999). Spanish researchers sought out underground oil-bearing areas in 2000 via the delineation of lithologic alterations and thermal anomalies caused by oil percolation (Gu et al., 2010). Chinese scientists successfully developed airborne hyperspectral the remote sensing PHI (Push-broom Hyperspectral Imager) and OMIS (Operative Modular Imaging Spectrometer) instruments in the 1990s, which have also been used in research applications (Yang et al., 2000; Zhou, 2014; Liu et al., 2016). Regarding soil adsorption, geochemistry, geothermal anomalies, and other factors, Chinese researcher sought remote sensing indications after systematically summarizing hydrocarbon percolation (Wang, 2010; Chen et al., 2007). Tian et al. determined the spatial distribution of oil percolation based on the oil and gas percolation of Inner Mongolia's Dongsheng area (Tian et al., 2000; Richards, 2005; Liu et al., 2011; Liu et al., 2015), using the diagnostic of mineral alteration absorption characteristics and field measurements of spectral curves (Kruse et al., 2012). Ni et al. used hyperspectral data to classify the natural gas alteration anomalies in an area of China and effectively extracted regional surface gas alteration characteristics with feature extraction based on the principal component analysis (PCA) wavelet method (Xu et al., 2007; Ni et al., 2007).
The general development of oil and gas applications of remote sensing technology in China and overseas indicates that hyperspectral remote sensing may be a favorable method for exploring new types of oil and gas pools (Goetz, 2009; White et al., 2009; Agar and Coulter, 2007). Research has demonstrated many reliable means of identifying additional oil reservoirs based on reflectance spectrometry and oil percolation theory, which have great significance for oil exploration (Wang et al., 2011).
Regional Geological Conditions
The Ordos basin is a large oil and gas field, enriched in Paleozoic coal-derived gas (Zhang et al., 2009; Wang et al., 2014; Zheng et al., 2006). Sugeli, Yulin, and other massive large-scale coal-derived gas fields have been identified (Yao et al., 2013; Zhu et al., 2013; Wang and Wang, 2013). The basin covers areas ofthe Shaanxi, Shanxi, and Gansu provinces and two autonomous regions, Ningxia and Inner Mongolia, covering an area of approximately 37x104 km2 in total (Wang et al., 2013; Li et al., 2010; Liu et al., 2008). The basement is a metamorphic rock series formed in the Archean to Paleoproterozoic. In the Mesoproterozoic to Neoproterozoic, it changed to a faulted trough that was filled with shallow sea classic rocks and carbonate sediments (Wang et al., 2008; Hao et al., 2012). The distribution of oil and gas and the structure of sedimentary caprock were controlled directly by the pattern of the basement tectonics (Liu et al., 2008; Luo, 2008; Duan et al., 2008). Then, in the early Paleozoic, it evolved to an infracratonic basin, and the sediment derived from an epicontinental carbonate platform. Since the late Paleozoic, a craton depression basin and a large inland depression basin successively formed due to lake and river sediment and eventually deposited thick loess in the Cenozoic era (Wu et al., 2015; Liu et al., 2006; Ren et al., 2006; Zhang et al., 2013).
As a multicycle superimposed basin, the ample petroleum resources of the Ordos basin provide a wide realm of bright prospects for oil and gas exploration (Zhao et al., 2006; Wang et al., 2015). The general tectonic framework controls the formation of the depression and the distribution of depositional systems that are fundamental for reservoir formation conditions (Liu, 2007; Zhao et al., 2012). The southern part of the basin primarily produces petroleum, and the northern part produces gas (Figure 1). Oil production occurs in shallow areas of the basin, whereas gas production occurs in the deeper parts. Four sets of oil-bearing systems that belong to the stratigraphic-lithologic reservoir have been exploited (Deng et al., 2005). The oil-bearing reservoir systems are the sandstone of the Triassic Yanchang formation, the lower Jurassic Yan'an formation, the Ordovician Majiagou carbonate group, and the Permo-Carboniferous coal-gas bearing system. The basin is ideal for the realization of the coordinated development of oil and gas in China (Liu et al., 2003).
There are many instances of oil and gas leakage in the Ordos Basin. The leakage above the oil and gas reservoir is in the form of a continuous or intermittent belt and in the area of fault development, the anomaly is distributed along the extension direction of the fault as a strip or string of beads.
The landform in the survey area belongs to the Loess Plateau, providing a high degree of rich exploration, geological and geophysical data that can be used. The behavior of geochemical indices is unique in that area, so the experience gained from studying other areas can be put to full use. Geochemical indices include: acid-hydrolysis methane, acid-hydrolysis heavy hydrocarbon, heat release methane, carbonate, altered carbonate, Fe2+, Fe3+, and some other indices. We fully excavate the information of hydrocarbon information indicated by hydrocarbons under different surface occurrences, verify the hyperspectral anomalies, and provide detailed geochemical data for further air and ground integrated oil and gas exploration.
Methods
Remote sensing technology with imaging spectrometry creates an image with spatial, spectral, and radiation information, thus allowing spectral analysis technology to reveal features directly (Chen et al., 2016). Using hyperspectral remote sensing technology can extract hydrocarbon percolation information such as vegetation lesions, mineral alteration, and micro-seepage from hyperfine spectra (Ellis et al., 2001; Lennon et al., 2006). In this study, the philosophies of remote sensing and petroleum exploration are combined to thoroughly understand oil and gas accumulation for comprehensive evaluation and full-scale planning. The core technology of the study includes remote sensing data processing and analysis, interpretation of geological information, and geochemical analysis. This study uses hyperspectral remote sensing images to comprehensively extract oil and gas percolation in the work area in addition to other methods indicated below.
Abnormal surface mineral spectral results were synthetically determined by collecting hyperspectral remote sensing images and extracting percolation information through preprocessing based on the surface spectral characteristic identification method of alteration minerals and comparative analysis. Upon the completion of geochemical testing of typical areas and response relationship analysis between remote sensing and surface geochemical abnormalities, the study examined the accuracy of hyperspectral remote sensing. Together, geochemical methods and remote sensing establish an integrated system that provides comparable means for oil and gas exploration.
Wave spectrum testing and surface matter analysis includes spectral measurements, filtering, Principal Component Analysis, maximum noise component transformation, and curve filtering of transect data analysis (Cao et al., 2003; Lei et al., 2008; Clark et al., 2003). According to the hyperspectral anomalies, typical areas were selected for geochemical testing; primarily, the tests involved microbial geochemical exploration, headspace gas examination, acid and alkali hydrocarbon methods, and fluorescent spectrometry (Lo et al., 2015).
The specific technical concept is as follows: starting from the geochemical field of the survey area, the geochemical behavior, the background field characteristics and the anomaly manifestations of the research methods in the adjacent area and other similar areas were compared. By using multivariate statistical processing and anomaly separation technology, we extract the concentration anomaly, fabric anomaly, and variation characteristic information of geochemical indicators, while removing the false anomalies formed by interference factors, and conduct comprehensive anomaly analysis. According to the petroleum geological significance and multivariate statistical analysis results of each index (Table 1), we selected five indices-acid hydrolysis heavy hydrocarbon, alteration carbonate, heat release heavy hydrocarbon, top air heavy hydrocarbon, and Fe2+/Fe3+ as the main geochemical indicators.
Typical regional geochemical testing
The study area is located in the Minhe basin, the juncture of Fuxian and Ganquan counties of Shaanxi province. The area has unique topographic and geomorphic conditions and extensive vegetation (Chang, 2013). The geochemical exploration deployed two study blocks, A and B, the former in Fuxian County and the latter in Ganquan County. This study focuses on the Fuxian area. The main methods used include the analysis of acidolytical hydrocarbons, pyrolysis hydrocarbon carbonate, carbonate alteration, and ferric ions. After analyzing the validity of the geochemistry index, the study focused on the capacity of bearing oil and gas in key target areas and anomaly points, and verifying the hyperspectral anomalies. All of those indices are needed to protect and explore oil- and gas-bearing areas. Moreover, it is necessary to fully tap the potential indicated by hydrocarbons near the ground surface for oil and gas resources and to verify the data obtained from hyperspectral remote sensing images.
After comparing the geochemical field of the study area and adjacent areas, the geochemistry index, background field characteristics, and abnormal appearances were studied. The analysis included multivariate statistical methods and other technologies that have been developed in recent years and have been used for anomaly separation. Conjunctive use of these technologies can lead to accurate assessments of favorable oil- and gas-bearing sectors while eliminating false anomalies caused by interference factors. After a study of the geochemical anomalies over oil and gas reservoirs, the response relationship between the oil field and geochemical anomalies was estimated, and the geochemical correspondence and distinction marks of oil and gas reservoirs were established both inside and outside the reservoirs.
Geochemical characteristics
The characteristics of those indices are displayed in Figure 2. The carbonate geochemical index presents a chaotic distribution compared with the distribution of a known oil field (Jiang et al., 2013) (Figure 2a). The methane and heavy hydrocarbon acidolytical indices mainly show a distribution area in the northeastern part of the study area. The pyrolysis hydrocarbon also reflects a high value in the northeastern part, consistent with the distribution of a known oil field (Figure 2b, 2c). The headspace index of the geochemical field of methane and heavy hydrocarbons have a different distribution, with high values of methane mainly occurring in a circular formation in the northeastern part of the study area; the other concentration is in the southern part, with a sequential circular formation, while the northern part also contains high points (Figure 2d). The geochemical characteristics of ferrous and ferric iron show a distribution pattern around the known site of an oil field (Figure 2e).
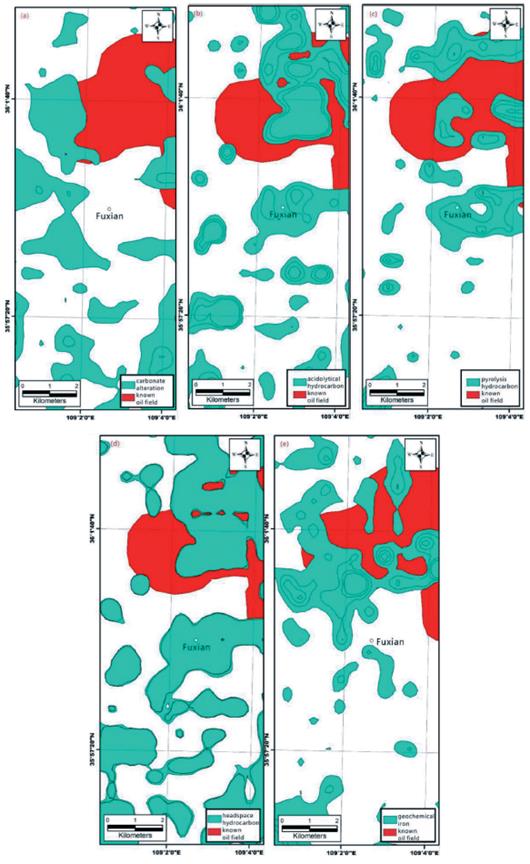
Figure 2 Geochemical anomalies (a. carbonate alteration, b. acidolytical hydrocarbon, c. pyrolysis hydrocarbon, d. headspace gas, and e. geochemical iron)
Through analysis of various indicators of geochemical fields, it is apparent that the index follows a certain regular variation. For instance, the pyrolysis and headspace heavy hydrocarbons show a high dispersion before iterations were conducted because of the multi-source superimposition of the latter stage. To highlight the geochemical anomalies and dispose of the interfering factors of non-oil and gas composition, pyrolysis and headspace heavy hydrocarbons were adopted as trend values, the values for acidolytical heavy hydrocarbon and carbonate alteration were used as concentration values, and single-index geochemical anomalies were applied to determine the ferric iron and ferrous iron values.
The response of remote sensing and geochemical information
Compared with the proportion of acidolytical heavy hydrocarbons, the pyrolysis heavy hydrocarbon, headspace heavy hydrocarbon, and hyperspectral anomalies are less than that for geochemical acidolytical heavy hydrocarbon in the study field, but remote sensing data has better correspondence with oil- and gas-bearing reservoirs (Figure 3c). The carbonate anomalies of the remote sensing data and the carbonate alteration of the geochemical method are basically consistent in southwestern Fuxian and show poor correspondence in the northeast (Figure 3a). The percent of coverage area for iron anomalies is good in the northeast (Figure 3b).
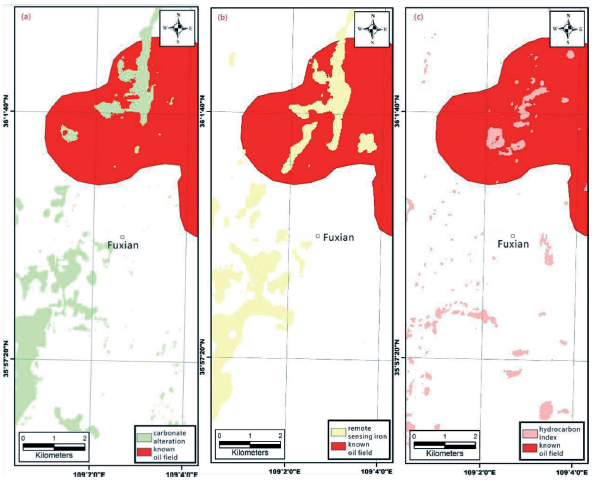
Figure 3 Hyperspectral remote sensing anomalies (a. carbonate alteration, b. iron anomalies, and c. hydrocarbon index)
Using special data processing technology, information regarding carbonate alteration, clay, iron-bearing mineral, and soil-absorbed hydrocarbons can be properly extracted, thus identifying abnormal areas quickly and providing a scientific basis for oil and gas exploration. In this study, different anomalies have different corresponding weight values. The proportion for the hydrocarbon anomaly is 0.3, clay is 0.25, carbonate is 0.25, and iron is 0.2. The value of the first-grade anomaly is greater than 0.7, the second-grade anomaly ranges from 0.5 to 0.7, and the third-grade anomaly ranges from 0.3 to 0.5. The study primarily considered first-grade remote sensing anomalies as the basis for assessment to determine prospective oil- and gas-bearing areas. Comprehensive anomaly analysis indicates that in southern Fuxian, the response relationship of geochemistry and remote sensing is not as ideal as expected, while the anastomosis degree is good in the north.
Comprehensive abnormality index
Due to numerous index properties and other interaction factors, different indices have their own genetic types, and the position of geochemical anomalies may be different. To improve the reliability of using geochemical methods to estimate oil- and gas-bearing reservoirs, it is necessary to use various methods and develop a comprehensive system that combines geochemical characteristics, the distribution pattern of high-value points, oil and gas traps, and other factors. The PI (Polarization index) uses average values, maximum values, and the key index effectively in abnormality analysis and assessment. It involves many factor estimates and an enormous amount of information; thus, comprehensive evaluation using the PI index better reflects actual geological conditions in anomaly analysis. For instance, it can indicate oil and gas information objectively (Yang et al., 2015).
A certain weight was assigned to each anomaly index: hydrocarbon anomaly 0.5, carbonate anomaly 0.3, iron anomaly 0.2. Values > 0.7 belonged to the primary anomaly, 0.5 to 0.7 to the second-grade anomaly, and 0.3 to 0.5 to the third-grade anomaly. Delineation of the far oil and gas scenic spots are mainly determined by the primary anomaly. The results show that in the area of the known reservoir, an anomaly belt shows an annular pattern with high strength and better continuity with a high degree of coincidence with a known reservoir (Figure 4a), and in another area, the anomaly points are located around the reservoir (Figure 4b). The interpreted results have good correspondence with the exploration results at this stage (Figure 4).
Remote sensing and geochemical comprehensive model
Various models for oil and gas exploration have been put forth based on dispersion migration mechanisms and oil and gas micro-percolation (Turner, 1980; Ungerer et al., 1984; Tissot et al., 2012; Blake, 2013). The reservoir hydrocarbons generate negative ions in the process of migrating upward along with fractions and produce a redox battery effect with anodic areas and anode surfaces because of the potential difference (He et al., 2013; Zhang et al., 2005; Lu et al., 2013). The hydrocarbon migration to the surface ground causes changes in the redox environment of different types of anomalies; for example, remote sensing images exhibited fading when the ferrous iron had been transformed to ferric iron (Wang et al., 2015; Luo, 2011). A wealth of data has demonstrated that geochemical anomalies are not controlled by a single factor because of the complexity of petroleum dispersity migration, and investigators have established a vertical percolation model of hydrocarbons that considers the factors of the migration mechanism, impetus, channels, and mineral alterations.
The research achievements above reflect the processes and results of hydrocarbon micro-percolation. Along with the migration path of fracture, joints, and bedding planes, light hydrocarbons migrate toward the surface in a vertical direction driven by many types of action, occurring in a series of geochemical, geophysical, topographical and landform anomalies in annular patterns above oil reservoirs. Using the available information, this study develops the oil and gas vertical percolation model in combination with comprehensive geochemical and remote sensing data (Figure 5).
Discussion and Conclusions
By processing and analyzing hyperspectral remote sensing and geochemical data, this study extracted percolation information concerning oil and gas reservoir exploration, and established a comprehensive model. The main research findings are as follows:
(1) Hyperspectral remote sensing anomaly extracting technology was established for petroleum geology and surface conditions through the collection of abnormal information of carbonate alteration, hydrocarbons, and iron-bearing minerals to ascertain abnormal prospective areas accurately.
(2) Geochemical blocks were deployed to collect samples for analysis of hydrocarbon percolation in the study area, and then, the response relationship with the reservoir was analyzed. The results demonstrated that the acidolytical hydrocarbon index is the most effective geochemical index, with a better correspondence with the oil field distribution compared with the headspace and carbonate alteration indices. Generally, hydrocarbon geochemical anomalies occur above the oil field as a continuous distribution of points that is consistent with oil reservoirs.
(3) Geochemical index and remote sensing data show that the hyperspectral exploration area coincides with the geochemical analysis at the surface and in the vertical profile. Comprehensive anomaly analysis indicates that in southern Fuxian, the response relationship of geochemistry and remote sensing are not as ideal as expected, while in the north, the degree of anastomosis is good.
(4) An oil and gas vertical percolation model was developed with a comprehensive combination of geochemistry and remote sensing data on the basis of available acidolytical hydrocarbon, pyrolysis hydrocarbon, carbonate alteration, and ferric and ferrous ion information.
The superposition of the geochemical index and remote sensing hydrocarbon anomaly shows that most of the planar hyperspectral remote sensing data are coincident with the geochemical exploration work area, and the longitudinal profile correspondence is more significant. However, there are also some regions, remote sensing anomalies, and geochemical indicators that do not show abnormal characteristics, so further studies are needed.
Combining remote sensing results with other non-seismic techniques not only provides new insight into the microleakage phenomenon, but also adds even more value to existing exploration data and increases the success rate of subsequent drilling.
A complicated composite relationship of remote sensing hydrocarbon anomalies with known oil and gas reservoirs was developed. In some regions, good anastomosis indicates that hyperspectral data provides great practicability for oil exploration. However, in other remote sensing anomaly areas, oil and gas reservoirs were lacking; therefore, further investigation is necessary.