Introduction
The production of various chromium compounds such as potassium dichromate and chromium trioxide is characterized by backward technology, small scale and scattered layout. Chromium pollution accidents occur frequently, resulting in a large number of water-soluble chromium Cr (VI) slag, which has seriously threatened human health and caused serious environmental pollution. Chromium enterprises have gradually become the focus of the government and all walks of life. For the purpose of source control, a large number of seriously polluted chromium enterprises have been shut down in recent years, and most of the chromium slag has been reasonably treated by the end of 2012 (Jourdan et al., 2018).
However, due to a large number of abandoned sites of closed chromium production enterprises have not taken necessary environmental protection measures, chromium residue has been piled up for a long time, and a large number of Cr (VI) has entered into the soil through the effect of wind and rain, causing the soil chromium content to exceed the standard seriously, and chromium contaminated soil has become a key problem of soil pollution. The survey found that there were more than one third of the total determination points, about 271 points, and chromium exceeding standard was one of the important indicators (Ojanen et al., 2018). After investigation, the pollution characteristics of excessive chromium in soil are as follows: from the analysis of valence state, the content of Cr (III) in soil chromium pollution is significantly higher than that of Cr (VI), the content of Cr (VI) in chromium residue site is only more than ten percent of Cr (III); from the analysis of morphology, the proportion of residual chromium is the largest. In reference (Cleveland & Townsend, 2019) suggests that the increase of nutrients in tropical rainforests will lead to the loss of a large amount of carbon dioxide from soil to the atmosphere. The terrestrial biosphere atmosphere carbon dioxide (CO) exchange is mainly in tropical forests, where the absorption of photosynthetic carbon (c) is considered to be limited by phosphorus (P).Phosphorus can also limit the decomposition of organic matter and the loss of soil carbon in Phosphorus Deficient tropical forests. The effects of phosphorus fertilizer on soil respiration in Costa Rica lowland tropical rain forest were studied through field fertilization experiments. In the early stage of wet season, when the input of soil soluble organic matter was large, the soil respiration rate increased significantly with the application of phosphorus. The results showed that the mechanisms driving the observed respiratory responses to nitrogen and phosphorus increase may be different, but nitrogen and phosphorus fertilization stimulated a large amount of CO loss. In reference (Jurkevitch et al., 2019), the characteristics of predation range, ribotyping, soil and Rhizosphere diversity of Bdellovibrio isolated from plant pathogenic bacteria were proposed. Thirty strains of Bdellovibrio were isolated from agricultural soil and plant rhizosphere. By means of molecular and culture methods, it was found that Bdellovibrio in soil contains different biological subpopulations from that in rhizosphere. 13 strains of soil and 7 strains of Soybean Rhizosphere Bdellovibrio were isolated from Pseudomonas corrugata, and 7 strains and 2 strains of soil were isolated from Erwinia carinii subsp. By analyzing the predation range of 5 isolates of Bdellovibrio in soil and rhizosphere, it was found that most of the 22 substrate species were plant pathogens and plant growth promoting bacteria, which had unique utilization patterns and differences among closely related strains. Although some progress has been made in the above research, there are some limitations in the remediation of high concentration chromium contaminated soil. Therefore, it is proposed to strengthen the research on the remediation of high concentration chromium contaminated soil by electrodynamic method.
Remediation of High Concentration Chromium Contaminated Soil by Enhanced Electrodynamic Method
There are two main ways to remediate chromium contaminated soil: A. remove chromium from the soil to make the retained concentration of chromium close to or reach the soil background value; B. change the existing form of chromium in the soil, reduce hexavalent chromium to trivalent chromium, and reduce its migration ability and bioavailability in the soil (Watson et al., 2019; Zehe et al., 2018; Walker et al., 2018). The removal of chromium from soil mainly focuses on chemical leaching, microbial leaching and phytoremediation. The optimization of chemical leaching agents is the research focus of chemical leaching technology, and the research and development of high-efficiency environmental protection chemicals is the future development direction. Citric acid, EDTA, GLDA and other chemicals used in the chemical leaching of chromium contaminated soil show good removal effect, and even clean water can effectively remove hexavalent chromium in soil (Rinella & Reinhart, 2020; Ser et al., 2020). Microbial leaching uses organic acids or surfactants produced by microorganisms to acidify or complexate with chromium to separate it from soil. Compared with chemical leaching, the cost of microbial leaching can be reduced by about 80%, which has little disturbance to the environment and will not cause secondary pollution. Therefore, it has been widely concerned in recent years (Shi et al., 2018; Zehe et al., 2018). Some scholars have successfully leached 52% - 68% of Cr from heavy metal contaminated soil by autotrophic microorganism Thiobacillus. Heterotrophic microorganisms such as bacillus, Pseudomonas and mold also have a good leaching effect on CR. At present, there are few studies on the removal of chromium from soil by microbial adsorption, mainly due to the difficulty of separation from soil (Zohdi, 2018; Frey et al., 2019; Marchiori et al., 2019). There are many studies on the remediation of heavy metal contaminated soil by hyperaccumulation plants, but there are relatively few studies on the removal of chromium. The research on stabilization of chromium contaminated soil mainly focused on chemical stabilization and microbial stabilization. Chemical stabilization technology has been widely used in the remediation of chromium contaminated soil, and the current research focus has gradually shifted from the optimization of chemical agents to the long-term safety assessment of the soil after remediation (Frey et al., 2019; Wang et al., 2018). The research on microbial stabilization began with the discovery of a Cr (VI) - reducing Pseudomonas strain in the 1970s. At present, dozens of genera including bacteria, actinomycetes, fungi and molds have been found to have Cr (VI) reducing ability.
Combined with other technologies. There are many cases of electric technology combined with other technologies to repair contaminated soil, mainly in the following ways: A. electric technology combined with PRB. The principle of this technology is to transform Cr (VI) into Cr (III), and the research content is to fill PRB medium with zero valent iron and ferric oxide (Nan & Yan, 2019; Sakae et al., 2018; Heckmann et al., 2018). The results show that the latter is better; B. Ultrasonic enhanced electrokinetic method can improve the migration ability of pollutants without any substances (You et al., 2018). At present, this method is often used to remove heavy metals from the soil; C. electrokinetic and biological (plants, microorganisms) joint remediation of chromium residue contaminated soil. Direct current was applied to make Indian mustard absorb heavy metals in the soil polluted by compound heavy metals (Yang et al., 2019); D. Using this technology to remove total petroleum hydrocarbons in soil, it is found that the removal rate of total petroleum hydrocarbons by electrokinetic method alone is 55%, and the removal rate of total petroleum hydrocarbons by Fenton electrokinetic method combined with iron electrode is 97%.
Materials and Methods
Cr(III)Preparation of contaminated kaolin
The experimental kaolin was purchased from the superfine calcined kaolin of Datong Jinyuan kaolin Co., Ltd. Accurately weigh 6.00kg of the kaolin in the polyethylene box; weigh 15.37g of chromium trichloride hexahydrate, dissolve and fix the volume to 2.00l volumetric flask, then add the solution and 2L deionized water into the polyethylene box with kaolin, mix evenly and balance for one week.
Chromium residue contaminated soil
Before the use of the soil, the chromium residue collected from a certain place in Henan Province by the laboratory polluted the soil. After air drying, the gravel and other debris were screened out, and the soil was powdered by ZN-08L small-scale pulverizer, and then the tested soil samples were screened through a 100 mesh screen for standby.
The basic properties of chromium contaminated soil are shown in Table 1.
Table 1 Physical and chemical properties of chromium residue contaminated soil
Physical and chemical unit | pH | Conductivity(mS/cm) | Saturated water content (%) | W(Cr(T))(mg/kg) | W(Cr(VI))(mg/kg) |
Size | 9.3-10.2 | 6.1-8.5 | 40 | 29291±50 | 4980±50 |
It can be seen from table 1 that the total chromium concentration of the tested soil sample is especially high, nearly 30000 mg/kg. Chromium mainly exists in the form of Cr (VI) and Cr (III), accounting for 17% and 83% respectively. By using the improved BCR extraction method, the proportion of various forms in the tested soil samples is 25.1% in acetic acid extractable state, 5.5% in reducible extractable state, 13.7% in oxidizable state and 55.7% in residual state.
Experimental device
The electrokinetic oxidation and electrokinetic PRB experiments were carried out by using the electrokinetic device. In the electrokinetic PRB experiment, a PRB medium with a width of 5cm was added at 5cm away from the anode. The device is made of organic glass, which mainly includes the following three parts: anode electrolysis chamber, cathode electrolysis chamber and soil chamber. The size of anode and cathode electrolysis chamber is: Length X w idth X he ight = 20 cm X 1 0 cm X 1 0 cm, and the size of soil chamber is: Length X width X height = 3 0 cm X 2 0cm X 1 0 cm . The same rod-shaped graphite electrode and electrolyte were placed in the anode and cathode electrode chambers, and the soil samples were evenly placed in the soil chamber. In addition, the device is connected with dc power supply (adjustable voltage), ammeter and peristaltic pump. The alkali solution tank and acid solution tank continuously add sodium hydroxide and acetic acid solution to the cathode chamber through peristaltic pump to balance the experimental pH; the 250 mesh filter screen is mainly used to block the electrode chamber and soil chamber to prevent soil from entering the electrode chamber; the collection tank is mainly used to collect anode and cathode electrolyte during and after the electromotion.
Instruments for experiment
The instruments and equipment required for the experiment are shown in Table 2:
Table 2 Instruments and equipment required for experiment
Serial number | Name of instrument and equipment | Model |
---|---|---|
1 | Electric experimental device | 30x20x10cm plexiglass column |
2 | Ammeter | 1~500mA |
3 | Digital acidity meter | FE20 |
4 | Conductivity meter | S230 |
5 | Electrothermal constant temperature blast drying oven | DH-101-3 BS |
6 | High speed centrifuge | LG10-2.4A |
7 | Atomic absorption spectrophotometer | AA6300 |
8 | Electronic balance | AL204 |
9 | Peristaltic pump | BT100-2J |
10 | Electric heating plate | EG20A Plus |
11 | Magnetic heating stirrer | 78-1 |
12 | Thermostatic oscillator | HZ-9511K |
13 | Small crusher | ZN-08L |
14 | Visible spectrophotometer | 722SP |
15 | Electronic scale | UWA-K015 |
16 | Ultraviolet visible spectrophotometer | 752 |
Experimental process
Batch test of potassium permanganate oxidation of Cr (III): the batch test was carried out in a 100ml conical flask with stopper, in which 100ml CrCl3»6H2O of certain concentration was added, the pH value was adjusted (0.1mol/l HCl and NaOH were used to adjust the pH value), and then a certain amount of KMnO4 was added. Put the conical flask into the shaking table (rotate speed 110R / min) to vibrate the reaction, take samples regularly, and determine the absorbance of Cr (VI) generated by oxidation.
Fenton reagent oxidation of Cr (III) batch test: the oxidant was replaced by Fenton reagent, and the others were the same as potassium permanganate oxidation of Cr (III).
Electric oxidation enhanced repair experiment: the 250 mesh filter cloth was fixed on the inner side of the porous filter screen of the reactor with a long tail clamp, and then the balanced 5 mesh filter cloth was accurately weighed with an electronic scale Kgcr (III) soil preparation or treated chromium slag contaminated soil was placed in the soil sample chamber of the electric device, the overflow port in the electrolysis chamber was blocked, 0.1mol/l KCl solution was added into the anode and cathode electrode chambers, and a certain concentration of potassium permanganate solution was continuously added into the cathode by using a circulating pump according to the conditions. After placing the electric soil and electrolyte, insert the carbon rod electrode connected to the digital display power into the electrolyte, power on, and apply a DC voltage of 1vgcm at both ends of the soil sample. During the test, acetic acid solution was dripped into the cathode electrode chamber by peristaltic pump to control the pH of the cathode solution to be acidic, and the current was recorded at intervals. After four days of electric driving, the electrolyte and soil samples were collected, and the soil samples were divided into 10 parts according to figure 1. Take 100-200g of each sample for drying, grinding, passing through 100 mesh sieve, and determine pH, conductivity, Cr (VI) and total chromium.
Electric PRB enhanced remediation of chromium residue contaminated soil experiment: 5 kg of chromium residue contaminated soil was accurately weighed by electronic scale after grinding and screening, put into polyethylene container, added 1.12 mol / L citric acid and deionized water, stirred evenly, acidified for about 4 days. 5 cm thick PRB medium was added into the soil chamber 5 cm away from the anode. 3.5 kg chromium slag was added to the contaminated soil in other parts of the soil chamber, and 0.1 mol / L KCl solution was added into the electrolysis chamber. After 9 days, samples were taken as shown in Figure 2 to determine pH, conductivity, Cr (VI), total chromium and other indicators.
Accurately weigh 4G of soil sample in a 100ml beaker, add 50ml of certain concentration of potassium chloride, conduct electromagnetic stirring for 5min, centrifugation, pour the supernatant into a volumetric flask of certain volume, repeat the above steps, combine the supernatant and fix the volume. The concentration of Cr (VI) in solution was determined by diphenylcarbazide spectrophotometry (GB 7467-87), and Cr (VI) in soil was calculated.
Take 100 mesh 0.5000 g (accurate to 0.0001 g) soil sample, put it in a polytetrafluoroethylene crucible, digest it with hydrochloric acid, nitric acid, hydrofluoric acid and perchloric acid on the electric heating plate, transfer it to a 50 ml colorimetric tube, add 5 ml 10% ammonium chloride solution, fix the volume, shake well, dilute, and inject the sample to determine the total chromium content. Take 10g soil sample into centrifuge tube, add 25ml deionized water, mix well, stand still, use digital acidity meter to measure the pH of solution.
After several days of electric driving, the following formula is used for calculation:
In the formula, represents the power consumption of electric repair (kWh), represents the voltage (U), represents the current intensity (A), and represents the operation time (h).
Take 5g soil sample into centrifuge tube, add 25ml deionized water, mix well, stand still, and measure soil conductivity with conductivity meter.
The batch experiments of potassium permanganate and Fenton oxidation of Cr (III) were carried out in order to select suitable oxidants and oxidation reaction conditions. The experimental conditions include reaction pH, time, temperature, oxidant concentration, etc. the reaction kinetics of potassium permanganate oxidation of Cr (III) was also studied. On this basis, the electrokinetic oxidation enhanced repair experiment was carried out.
Analysis of experimental results
The effects of pH, time, temperature and oxidant concentration on the oxidation of Cr (III) were studied by the reaction of 50.00 mg/L Cr (III) with a certain concentration of potassium permanganate. The reaction kinetics of Cr (III) oxidation by potassium permanganate was also studied.
Effect of pH on oxidation of Cr (III) by potassium permanganate
At relatively high pH, permanganate reacts to form manganese dioxide, which is expressed as follows:
Potassium permanganate can react to form Mn2 + at lower pH. the reaction equation is as follows:
When pH is higher than 12, the following reactions will occur:
It can be seen that potassium permanganate has strong oxidizability under acidic conditions. In addition, when pH is higher than 7, Cr (III) will exist in the form of precipitation, which makes it more difficult for potassium permanganate to oxidize Cr (III). Therefore, in order to study the optimum pH for the oxidation of Cr (III) by potassium permanganate, the range of pH greater than 7 was directly omitted, and the experimental pH range was set as 1 ~ 7. The traditional electrokinetic remediation technology only migrates and concentrates the mobile target pollutants into the electrolytic cell, and the removal efficiency of most of the difficult to migrate Cr (III) in the chromium residue contaminated soil is low. Therefore, in order to improve the removal rate of Cr (III) and total Cr in the soil polluted by chromium slag, and to repair the soil polluted by chromium, the actual soil polluted by chromium slag was selected as the test soil. Two enhanced electrokinetic remediation methods, electrokinetic oxidation enhanced remediation technology and electrokinetic PRB enhanced remediation technology, were studied in order to provide technical support for enhanced electrokinetic remediation of chromium residue contaminated soil.
A. A series of batch tests were carried out to select the suitable oxidant and the optimum oxidation reaction conditions. The effects of pH, time, temperature and oxidant concentration on the oxidation of Cr (III) by potassium permanganate and Fenton reagent were studied.
B. Potassium permanganate oxidation enhanced electrokinetic remediation of Cr (III) contaminated kaolin was carried out, in which the prepared kaolin Cr (III) concentration was 500 mg • kg-1. The experiment mainly studied the feasibility of potassium permanganate enhanced electrodynamic chromium removal, and analyzed the current, pH, soil moisture content, potassium permanganate concentration and other factors.
C. The experiment of potassium permanganate oxidation enhanced electrokinetic remediation of chromium residue contaminated soil was carried out to study the changes of pH, moisture content, Cr (VI) and total chromium of chromium residue contaminated soil after electrokinetic oxidation.
D. In order to further study the mechanism of enhanced remediation of chromium residue contaminated soil by electrokinetic PRB, Fe3O4, Fe3O4 + activated carbon, Fe3O4 + hydroxy alumina were used as PRB media, respectively.
The test results are shown in Figure 3.
It can be seen from Figure 3 that under acidic conditions (pH = 1.0-6.0), the amount of Cr (VI) is more and the difference is not big. The highest Cr (VI) concentration was 47.4 mg / L at pH 3.0. The oxidation rate of Cr (III) reached 94.8% from the initial Cr (III) concentration of 50.00 mg / L before the reaction and the conservation of element mass. The lowest Cr (VI) formation rate was found at pH 6 in acid condition, but it also reached 41.55% The results show that the oxidation rate of Cr (III) is 83.1%, and the Cr (VI) concentration is 32.33 mg / L under neutral condition. Therefore, the optimal pH of the experiment is 1-6. Although the H + generated at pH 6 may be oxidized by permanganate to increase the consumption of potassium permanganate, considering the economy, the formation rate of Cr (VI) and the follow-up experiment, the follow-up experiment chooses pH 6.
Effect of different initial Cr (III) concentration on oxidation
Four different initial concentrations of Cr (III) (10.00 mg/L, 20.00 mg/L, 35.00 mg/L, 50.00 mg/L) were set to react with 2 g/L potassium permanganate at pH 6, temperature 20 °C and rotating speed 120 R/min. The experimental results are shown in Figure 4.
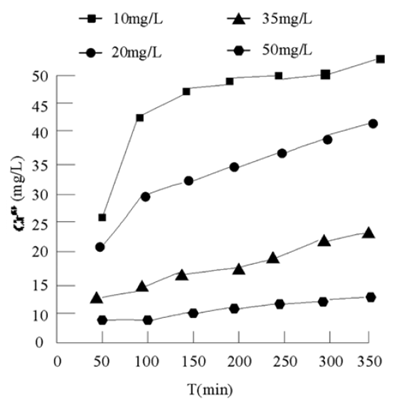
Figure 4 Variation of the amount of Cr6 + generated by Cr3 + at different initial concentrations with time
It can be seen from Figure 4 that with the progress of time, the formation of Cr (VI) in four different reactions is significantly accelerated in 0 ~ 60 min, that is, Cr (III) and potassium permanganate react rapidly in this period. After 60 min, the amount of Cr (VI) increased, but the change was not obvious. At this time, the reaction rates of the above four different initial Cr (III) concentrations were more than 80%. Therefore, the sample was taken at 60 min in the follow-up experiment.
The kinetics of potassium permanganate oxidation of Cr (III) was studied. Different sampling time (0, 5, 10, 20, 25, 30, 45, 60 min) was set in 0-60 min. the sampling analysis results are shown in Figure 5.
It can be seen from Figure 5 that the Cr (VI) concentration gradually increases with the reaction. The pseudo first-order dynamic simulation was carried out during this period. The oxidation of Cr (III) by potassium permanganate followed pseudo first order kinetics under the condition of 2g / L potassium permanganate, the results are shown in formula (5):
In the formula, Cr3+ is the concentration of Cr (III) at time t, that is, Ct. Therefore, R2 and kobs (pseudo first order kinetic constants) can be obtained by plotting ln Ct against time T. The results are shown in Table 3.
Table 3 Pseudo first order kinetic parameters of potassium permanganate oxidation of Cr (III)
Initial CR 3+ concentration | 10mg/L | 20mg/L | 35mg/L | 50mg/L |
Kobs | 0.035 | 0.038 | 0.098 | 0.205 |
R2 | 0.924 | 0.969 | 0.966 | 0.965 |
It can be seen from table 3 that when Cr (III) concentrations are 10.00 mg / L, 20.00 mg / L, 35.00 mg / L and 40.00 mg / L respectively, adding potassium permanganate with concentration of 2G / L, the pseudo first-order kinetic constants of the reaction are small, but they slowly increase, and the R2 of four different initial Cr (III) concentrations are all above 0.9. Therefore, the pseudo first order kinetics can be used to describe the reaction of Cr (III) with excessive potassium permanganate.
In order to further study the effect of temperature on the oxidation of Cr (III) by potassium permanganate, four different temperatures of 10 °C, 20 °C, 30 °C and 40 °C were set. 50 mg/L Cr (III) was oxidized by 2 g/L potassium permanganate. The reaction was carried out at pH 6 and 120 R / min. samples were taken at different intervals. The results are shown in Figure 6.
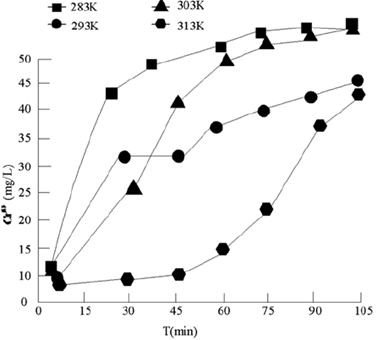
Figure 6 Variation of Cr (VI) formed by oxidation of Cr (III) by potassium permanganate with time at different temperatures
Temperature has a great influence on the oxidation of Cr (III) by potassium permanganate. It can be seen from Figure 6 that at 313 K, the reaction end point is close to 10 min. in comparison, at 283 K, the reaction begins to approach the end point after 90 min. That is to say, the higher the temperature is, the more favorable the reaction is. Therefore, the oxidation of Cr (III) by potassium permanganate is an endothermic reaction.
Enhanced remediation of chromium residue contaminated soil by electrokinetic PRB
Because of the wide source and low price of aluminum salt, and through the experiment of adsorption of Cr (VI) by hydroxy alumina, we know that hydroxy alumina has a strong adsorption performance for Cr (VI). Therefore, hydroxyl alumina can be used in PRB medium in the experiment of enhanced remediation of chromium contaminated soil by electrokinetic PRB.
In order to further study the method of enhanced electrodynamic chromium removal and the specific mechanism of electrodynamic PRB enhanced remediation, the design conditions of electrodynamic remediation for a chromium residue contaminated soil in Henan Province are shown in Table 4. The length, width and height of the filled PRB medium are about 20 cm, 5 cm and 10 cm respectively.
Before electrokinetic remediation, the pH value of chromium residue contaminated soil was above 9.5. According to the experimental conditions of electrokinetic PRB enhanced remediation of chromium residue contaminated soil as shown in Table 4, after electrokinetic remediation for 9 days, the pH value of each part of chromium residue contaminated soil of three groups of electrokinetic PRB enhanced remediation decreased, ranging from 9.0 to 9.25. The specific distribution of pH in soil is shown in Figure 7.
Table 4 Experimental results of enhanced remediation of chromium residue contaminated soil by electric PRB
Number | Time/d | Voltage/V | Cathode liquid | PH control of anode and cathode | PRB media | Anode solution |
1 | 9 | 30 | 0.1M/L KCl | pH control test of NaOH / acetic acid | Fe3O4 | 0.1M/L KCl |
2 | 9 | 30 | 0.1M/L KCl | pH control test of NaOH / acetic acid | Fe3O4+Hydroxyl aluminum(Mass ratio 2:1) | 0.1M/L KCl |
3 | 9 | 30 | 0.1M/L KCl | pH control test of NaOH / acetic acid | Fe3O4+Activated carbon(Mass ratio 2:1) | 0.1M/L KCl |
Before electrokinetic remediation, the pH value of chromium residue contaminated soil was above 9.5. According to the experimental conditions of electrokinetic PRB enhanced remediation of chromium residue contaminated soil as shown in Table 4, after electrokinetic remediation for 9 days, the pH value of each part of chromium residue contaminated soil of three groups of electrokinetic PRB enhanced remediation decreased, ranging from 9.0 to 9.25. The specific distribution of pH in soil is shown in Figure 7.
The pH distribution in Figure 7 is mainly due to H+ produced by anode electrolysis. H+ moves to soil pores under the action of electroosmosis and electromigration, which makes the pH of soil near anode lower than that near cathode. Although Oh - is produced by cathode electrolysis, the pH of electrolyte is always acidic due to the existence of pH control system, which makes most of OH- produced by cathode neutralize with acetic acid, so that the pH of soil near cathode does not exceed the initial pH of soil. In addition, the pH control system makes the pH of anode and cathode electrolyte always maintain acidic, and the migration speed of H+ is faster than that of OH-, but the pH of the soil is still alkaline after electrokinetic, which is mainly due to the strong alkaline of the tested soil itself.
Conclusions and Prospect
A. Based on the results of oxidation of Cr (III) by potassium permanganate and Fenton reagent, the amount of Cr (VI) produced by Fenton reagent is only about 9 mg / L when the initial Cr (III) is 200 mg/L and the condition is relatively suitable, which shows that the ability of Fenton reagent to oxidize Cr (III) is poor. Relatively speaking, when the initial Cr (III) concentration is 50 mg / L, 20 °C, pH is 6, potassium permanganate is 2g/L, and the reaction time is 60 min, the Cr (VI) production has reached about 45 mg/L, and the removal rate is as high as 90%. Therefore, potassium permanganate is selected as the oxidant in the subsequent electric remediation of chromium residue contaminated soil.
B. The oxidation of Cr (III) by potassium permanganate occurred under acidic conditions. When the pH was 3, the concentration of Cr (VI) was 47.4 mg/L, and the oxidation rate of Cr (III) was 94.8%;
C. The oxidation of Cr (III) by potassium permanganate is greatly affected by the concentration and temperature of potassium permanganate. With the increase of potassium permanganate concentration and reaction temperature, the formation of Cr (VI) first increases and then tends to be stable. The reaction between potassium permanganate and Cr (III) is a pseudo first order reaction depending on the concentration of potassium permanganate. When the concentration of potassium permanganate is not excessive, the reaction kinetics conforms to the second order kinetics The pH control system makes the pH of anode and cathode electrolyte always keep acidic, and the migration speed of H+ is faster than that of OH-, and the pH of soil near anode is lower than that near cathode.
Enhanced electrodynamic method can improve the removal efficiency of total chromium to a certain extent, but there are also some problems as follows
A. Potassium permanganate added to the soil will have a certain impact on the physical and chemical properties of the soil, and damage the quality of the soil to a certain extent. Therefore, what kind of soil is suitable for electrooxidation technology and how to properly treat the soil added with potassium permanganate after electrooxidation are worthy of further study.
B. In the process of electrooxidation, there is still a lot of research space in the form of Cr (III) oxidized by potassium permanganate and the change of soil Cr form after electrooxidation.
C. In the electrokinetic test, Fe3O4, hydroxyl alumina and activated carbon are used as PRB media, how long the repair effect can last, and it needs to increase the electrokinetic time for further research; when the PRB media fails, how to regenerate and realize the recycling of the media is also a direction of further research.