1. Introduction
Construction is one of the essential industrial activities for the development and progress of cities. However, it is also one of the sectors that contributes most to environmental impacts, due to the extraction of raw materials, energy use, and waste generation.
It is considered that the construction sector consumes more raw material and energy than any other economic activity, and generates the largest fraction of waste. In Europe alone, around 900 million t of construction and demolition waste are produced every year (Bravo et al., 2015).
According to Ramesh et al. (2010), the term construction and demolition waste (CDW) refers to solid waste produced in the construction sector. More specifically, the term is defined as the waste that arises from construction, renovation and demolition activities.
CDW includes a range of materials such as ceramic products, concrete waste and asphalt material, and to a lesser extent other components such as wood, glass and plastics (Yuan and Shen, 2011). The main components of this waste depend on the materials used, the construction practices, and the technological development of the sector.
There are various management options for this waste, whose hierarchy depends on the environmental impacts they generate. The five levels of low to high environmental impact are: reduction, reuse, recycling, incineration and final disposal. Some authors (Yuan and Shen, 2011) have grouped these six levels into four: waste reduction, reuse, recycling and disposal. However, the impacts of management systems vary. For example, inadequate disposal of construction and demolition waste (CDW) can generate negative environmental impacts such as soil degradation and erosion, destruction of vegetation, and loss of environmental services (Mejía et al., 2015).
The impacts of waste disposal in landfill are associated with the extraction or obtaining of raw material, and land occupation. If waste is sent to landfill, then new materials or products must be manufactured from original raw material (Suárez Silgado, 2017).
Faced with this situation, European countries have tried to find innovative alternatives for the recovery of waste from construction and demolition. Yilmaz et al, (2018) have used construction and demolition waste as cemented paste backfill material for underground mine openings. Also, numerous EU regulations have been drawn up on this topic. One is the European Waste Directive, which foresees that by 2020, 70% of CDW should be properly valued. The objective is to achieve much higher levels of recycling by minimizing the extraction of additional natural resources. Thus, prevention and recycling are key elements of the new waste policy in Europe (Suárez-Silgado, 2016).
In this same line, several studies have used life cycle assessment for effective municipal waste management, because it helps in environmental evaluations of alternative waste management systems (Koci and Trecakova, 2011). According to ISO 14040 (2006), LCA is composed of an inventory of the relevant inputs and outputs of the system, the definition of the goal and the scope, an assessment of the potential environmental impacts associated with these inputs and outputs and, finally, an interpretation of the results of the inventory and impact phases in terms of the study objectives. This methodology has been used by several prominent authors in the field, including Zabalza et al., 2011; Monahan and Powell, 2011 and Tosic et al., 2015.
Mercante et al. (2012), Coelho and de Brito (2012), Yeheyis et al. (2013), Carpenter et al. (2013), Dahlbo et al. (2015), Guignot et al. (2015) and Wang et al. (2018) have used the LCA for the environmental assessment of CDW management systems in Europe, North America and Asia.
The situation has led to the development and implementation of technologies in the international area. The CDW in China are usually randomly dumped or disposed in landfills and the average recycling rate of CDW is only about 5% (Huang et al., 2018). In countries such as Denmark, the Netherlands, and Belgium, the recycling of CDW for uses other than landfill is promoted, and recycling percentages of over 75% are achieved. This high level of recycling is mainly due to the shortage of natural aggregates and space for landfill sites (Zabalza Bribián et al., 2011). One factor that has increased recycling rates has been the increase in the cost of landfilling, or its prohibition in some cases, such as in Denmark or the Netherlands. However, in most South American countries this is not the situation. The management of CDW waste is carried out inadequately, and there is clearly a large gap between South American and other countries in terms of management and technology.
In Colombia, CDW is sometimes managed using a controlled discharge system, but usually its disposal is uncontrolled. The authorized sites for waste disposal are disseminated widely, and there are few alternatives for recovery, recycling or reuse. For this reason, only 5% of CDW is recycled in Colombia (Castaño et al., 2013).
The generation of CDW has great environmental implications in this country, as it is sometimes disposed of in illegal landfills or thrown on public roads contributing to changes in the landscape and urban areas (Aguilar et al, 2010; Pinzón, 2014).
The situation is particularly palpable in some municipalities in the country, such as Ibagué, where around 488.000 t of CDW are generated per year (Ibagué Limpia, 2017), without counting the waste that is generated and disposed of clandestinely. The problem has increased in recent years, due to population growth, increased construction activity, and remodeling of buildings. However, an attempt has been made to advance in this area, and for this reason regulations and programs have been promulgated at district level that encourage the adequate disposal of CDW. Examples are Resolution N°1115/2012, which technically regulates the treatment and/or use of CDW in the capital district; and the Municipal Development Plan "Bogotá Humana" (2012-2016) with the "Zero Waste-Rubble Zero" program.
In Ibagué, there have been new initiatives for CDW management, expressed in Agreement No. 19 of 2013. The Agreement implements Environmental Compare as a tool for citizens related to the proper management of solid waste in Ibagué, and the Integral Solid Waste Management Plan of Ibagué (PGIRS, 2015), which aims to promote the integral management of CDW, broaden the characterization of this waste, and design programs to take advantage of CDW through feasibility studies.
In the same field, studies have been carried out at national level on the perspectives and limitations of CDW management (Castaño et al., 2013; Pinzón, 2014), and on the current waste situation in some municipalities (Jiménez, 2013). Technical diagnoses have been made of the use of CDW in the capital district (Escandón, 2011; Chávez et al., 2014), along with studies of quantification and characterization of CDW (SDA, 2012; Pinzón, 2014). Finally, in some cases, pilot proposals for recycling plants have been made (Chávez et al., 2014).
However, despite these advances at national level, no studies have been undertaken to date on the environmental assessment of managing fractions of this waste. Therefore, to help solve the problem of CDW generation in Colombia, the objective of this study was to evaluate the current treatment or management of each fraction of CDW. Subsequently, other alternatives were evaluated in which either the treatment that is used for fractions of waste or the percentage of waste in the treatments was modified. Hence, the objective was to determine which is the most beneficial management alternative at environmental and economic level.
The area chosen for the study was the department of Tolima, specifically, its capital Ibagué. This area was selected for two main reasons: the current problems associated with the generation of CDW because of population growth and an increasing number of buildings, and the new waste management initiatives described in Agreement No. 19 of 2013 and the PGIRS (2015), mentioned above. The research is novel because the environmental impacts of waste management systems in Colombia were evaluated using life cycle assessment (LCA), to obtain a more realistic view of the impacts in this country, specifically in Ibagué.
As Life Cycle Analysis (LCA) is a decision tool, the results could help to encourage or give greater impetus to the development of projects and programs that contribute to CDW recycling or reuse.
The management scenarios were also evaluated from an economic perspective. In addition to applying LCA methodology, a sensitivity analysis was carried out to determine how the final results are affected. The results obtained may lead to the creation of more demanding regulations in the field of waste management in Colombia.
2.1 Study area
This study was carried out in Ibagué, a city located in the center-west of Colombia on the Central Mountain Range of the Andes, at an altitude of 1.285 m.a.s.l. This city has an extension area of 4.605 hectares, according to the Territorial Ordinance Plan (POT, 2015), and a population of 553.524 inhabitants (DANE, 2015). The average annual generation of construction and demolition waste is 488.000 t, according to data provided by Ibagué Limpia (2017). For this study, it is considered that waste is generated in the expansion zone, where the largest amount of waste is produced.
2.2 Description of the construction and demolition waste in the study area
In accordance with the regulations that apply in this country (Resolution 1115/2012), construction and demolition waste are generated during the development of a construction project, and include excavation products, leveling and leftovers from site preparation; products used for foundations and pilings; stone waste (concrete, sand, gravel, pieces of bricks and blocks, ceramics, leftovers of mortar and concrete mix); and non-stone waste (glass, wood, plastics, metals, cardboard and gypsum).
Construction companies in the city were visited to gather information about the current management of CDW in the city of Ibagué. Direct contact was also established through telephone calls and email. According to the information obtained from the companies, over 80% of them are engaged in constructing new dwellings. Therefore, the largest amount of waste in this sector comes from construction processes. Of all the construction activity, 92.8% corresponds to dwellings (ICER, 2016).
Figure 1 shows the composition of CDW in the study area. The highest percentage corresponds to excavated earth. The amount of excavated land that is generated is high compared to other countries. The reason is that in Colombia construction companies (in which the earth movement phases are notable) predominate over demolition companies. In another similar study, a high percentage of this waste was found in Ibagué (Suárez-Silgado et al, 2018).
The management system currently applied for CDW in the city of Ibagué (according to the information provided directly by construction companies in the city in 2017) consists basically of the generation of waste in construction, and its subsequent transport to the landfill or final disposal site, without any treatment or recovery. In some cases, around 50% of construction companies separate the metal and excavated earth from the rest of the waste. The metals that are separated are transported by managers to a metal classification or preparation plant for subsequent recycling in a smelting plant in another city. Excavated earth is separated to be reused in the same construction or on a nearby site.
In some construction projects, waste is not separated and is simply taken to landfill. This management system is due partly to the fact that Ibagué does not have a recycling plant for CDW, and therefore the waste is mostly taken to the final disposal site. However, in the absence of sufficient control and monitoring in the management of these sites, waste is also transported to non-formal disposal sites in some cases, causing great damage to public roads, riverbeds, and vacant lots. Exact data on this form of disposal are not known.
2.3 Life cycle assessment (LCA) methodology
2.3.1 Goal and scope definition
Considering the current management of CDW in Ibagué and the problems associated with waste generation in this city, the objective was to identify the potential environmental impacts associated with the management of construction and demolition waste fractions and compare them with new alternatives. The functional unit of the study was 1 kg of waste.
Based on the characterization of waste in Ibagué (Figure 1) and the information obtained from construction and other companies that were consulted directly, the three waste fraction considered were: stones (19.5%) (concrete, brick and other inerts), excavated earth (80%) (essentially sands and clays; the organic material content within the ground was considered negligible) and metals (0.5%) (steel).
2.3.2 Alternatives
Three alternatives were evaluated in the life cycle assessment (Table 1). They were chosen according to the quantity and characterization of the waste and the current waste management system in the study area; information that was provided by IBAGUÉ LIMPIA (2017).
The first alternative (A1) corresponds to the current management of waste in the city of Ibagué. In this alternative, all stone waste is taken to landfill, metals are taken to the sorting and compaction plant and 50% of excavated earth is reused on a nearby construction site (5 km), while the other 50% is taken to landfill.
In two alternatives (A2 and A3), the waste treatment and percentages were modified, to determine viability.
In alternative A2, the stone waste management was modified. In this case, 50% of stone is recycled and the other 50% is taken to landfill.
In alternative A3, 100% of all waste is recovered. All the stone is recycled and all the excavated earth is reused.
Figure 3 shows the limits of the study systems. The generation and separation of waste fractions were not considered, as waste is separated on site. Only the stages of transportation and waste management were included. Once the waste has been generated and separated, it is transported for treatment or final disposal. Thus, the type of management (recycling/recovery, reuse and disposal) varied depending on the waste fraction. Finally, the loads of processes that are avoided were subtracted.
2.3.3 Life cycle inventory
The aim was to obtain all data directly through company visits and incorporate them into the inventory. Data that could not be obtained in this way (above all, emissions data) were complemented with the Ecoinvent database. In all cases, data were contextualised for the Colombian context.
Information was obtained from primary sources through visits, telephone calls and emails to construction companies, CDW and metal recycling plants, inert landfill sites and quarries. Government organizations such as IBAGUÉ LIMPIA and CORTOLIMA were contacted. Fifty-six construction companies registered in Ibagué, the existing legal inert landfill, 4 metal recycling plants, and 6 quarries near the study area were also contacted.
As the city of Ibagué does not have a CDW recycling plant, data had to be obtained by visiting waste recycling plants that are currently in operation in Colombia. Data were also collected from other facilities at national level and from 2 landfills to complement and compare with data from landfills in the study area.
The data correspond to different years (2015-2017) with a similar production capacity. The information was supplemented with secondary sources such as articles, journals, projects on the same subject, and the Ecoinvent v3 database.
To evaluate the environmental impacts of each of the management processes applied to the CDW fractions in Ibagué, new processes were created in Ecoinvent v3, taking as a starting point the information collected from companies, recycling plants and landfill, completed with the inventory of the Ecoinvent v3 database. The electricity mix of Colombia was used for the calculations.
Each of the treatment or management processes was evaluated per kg of waste to obtain the environmental impacts. To determine the environmental impacts of managing 1 kg of CDW generated in Ibagué, a new process was created in which each treatment was incorporated, considering the percentage that it occupies in the total construction and demolition waste (Figure 2). In this way, each of the alternatives was compared.
Next, each type of treatment or management was defined: earth reuse, metal recovery (classification and compaction), stone recycling, stone and earth landfill.
• Excavated earth reuse
The process of reusing excavated earth was evaluated in another nearby construction site. It was assumed that the excavated earth was not contaminated and could therefore be reused without any other type of treatment. The external transportation to the other site is considered. According to the information obtained from the companies, 5 km was considered the maximum distance between the extraction site and the reuse site.
The compaction of earth on the new construction site and the emissions (leached) due to its use (100 years) were also considered.
Solid waste was considered as output, which is transported to a sanitary landfill located 11 km from the site on which the waste is generated.
The reuse of excavated earth on a nearby construction site avoids disposal of this material in landfill. Therefore, the costs of transporting excavated earth to the landfill and its disposal were subtracted in this case.
Table 1 shows the input and output of the reuse of excavated earth and the process that is avoided.
• Metal (steel) recovery
For the recovery of metal, the stage considered was transport to the treatment plant, where the waste is prepared (classified and compacted) before it is cast, since there is currently no foundry for this material in Ibagué.
It was assumed that waste comprised of steel is separated on the construction site and transported separately to the treatment site. The process in these plants basically consists of classifying the material and compacting it, for subsequent export and smelting. According to the companies consulted the steel waste is transported from the expansion area of Ibagué to the classification and compaction plants located at an average distance of 9 Km.
The production capacity of the metal treatment plant was taken to be approximately 3500 t/year of waste, with an infrastructure of 1500 m2.
As the recovery of this waste and its preparation for use as secondary raw material (scrap) replaces the production of iron ore, this production is classified as an avoided product, so the impacts due to this process can be subtracted.
Table 2 shows the inputs and outputs of the recovery of metal and the process that is avoided.
• Stone recycling
Taking into account the information collected and based on the existing unit processes in the Ecoinvent v.3 database, a process called stone recycling was identified. From the amount of CDW generated in the city of Ibagué (2017), it was determined that stone waste made up of 45% brick and 55% concrete.
For stone waste recycling, crushing of the aggregate was considered in a fixed recycling plant.
As there is currently no recycling plant in the city of Ibagué, a possible location was chosen, considering the map of the area and the Territorial Ordinance Plan (POT, 2015). A viable site would be suburban land in this city, which would allow the location and development of an industry of this type, since it is inside the industrial zone. The site chosen for a possible location of the recycling plant is 7.5 km from where the waste is generated (Ibagué expansion zone).
This distance is well below the limit proposed in Ulubeyli et al. (2017), which states that the distance between facilities should be no more than 50 km for them to be viable environmentally and economically. Based on these data, the t-kilometers to be entered in the Ecoinvent v3 database were calculated. It was found that 0.008 tkm is used to transport 1.02 kg of stone waste. It was assumed that ceramics and concrete waste had been separated from the rest of CDW in work.
The output of the recycling process was considered to be air and water emissions, the product of the crushing process, and the steel recovered from the reinforced concrete that is included in concrete and brick stone waste.
To calculate the amount of steel generated by mixed aggregate, it was assumed that 1 kg of concrete generates 0.02 kg of steel (NSR, 2010). As the mixed aggregate was composed of 55% concrete, according to data from IBAGUÉ LIMPIA (2017), then 0.55 kg of concrete generated 0.01 kg of steel. This value was taken as the maximum amount of steel generated from the mixed aggregate.
It was assumed that the generated steel waste could be transported to the treatment plants that are currently situated in Ibagué, which classify and compact the metal. Therefore, in this study, the steel management system included only transport to these plants. In this case, an average distance of 16 km was selected, according to the maps (POT, 2015).
The transport of aggregate extracted from the quarry was considered a process that was avoided. It was assumed that the recycling plant had a maximum production capacity of 100000 t/year.
Table 3 shows the inputs and outputs of stone recycling and the process that is avoided.
• Excavated earth and stone landfilling
The stages of landfill disposal are similar for stone and earth. This process considers landfill infrastructure, land occupation, fuel consumption for compaction and grading of the material, and the effect of landfilled waste (leachate).
In this process, it was considered that waste from construction works is transported from the expansion zone to the inert waste landfill located at 13 km. Factors that were considered included the landfill infrastructure (14 hectares), the use of land, and fuel (diesel) consumed in the compaction and grading of the material.
A life cycle inventory (LCI) for inert landfill rarely includes leachate, since in general the waste material in this kind of landfill has a low pollutant content, and is chemically inert to a large extent. However, according to Bovea et al. (2016), models for inert material landfills should take these emissions into account, since a small percentage of biodegradable materials may be disposed of at these sites. Considering studies by Butera et al. (2015) and Colomer et al. (2017), the leachate emissions framework was considered to be 100 years. The leachate composition from CDW landfill according to López and Lobo (2014), Bovea et al. (2016), and Ecoinvent v3 was taken into account. Table 5 shows the composition of the leachates in the inert waste landfill. The landfill gas collection system was excluded, since this was not relevant in a landfill for CDW (Butera, 2015; Bovea et al,, 2016).
The Table 4 shows the inputs and outputs of this process.
2.3.4 Life cycle assessment
For the study of the LCA, the impact categories were chosen based on several studies in this field. These studies are shown in Table 7. The impact assessment method selected for the study is IMPACT 2002+, which has been used by several authors (Hossain, 2016; Hossain, 2017; and Suárez et al, 2016). This method is one of the most feasible and widely used environmental impact assessment methodologies. It combines a midpoint and damage approach, and links all types of life cycle inventory results via several midpoint categories to several damage categories (Hossain, 2017).
Table 7 Impact categories evaluated
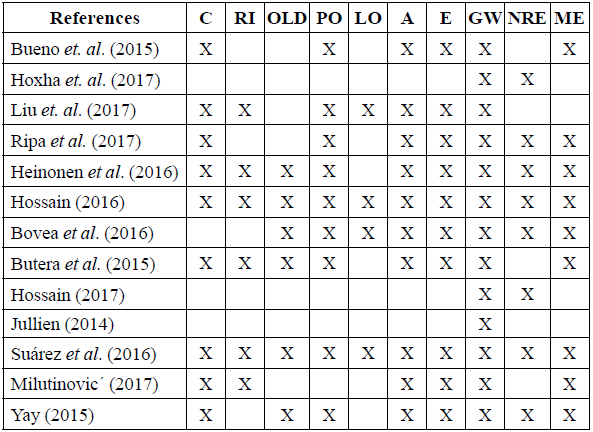
C= Carcinogenic; RI= Inorganic respiratory; OLD= Depletion of the ozone layer; PO= Photochemical oxidation; LO= Land occupation; A= Acidification; E= Eutrophication; GW= Global warming; NRE= Non-renewable energy; ME= Mineral extraction.
The software used to perform the calculations was SimaPro 8. SimaPro is one of the most widely used and accepted LCA tools. It helps to model various products and processes, and analyses the results to achieve sustainability goals. SimaPro includes many LCI datasets, including the renowned Ecoinvent v3 database, the new industry-specific Agri-footprint database, and the ELCD database (PRé Consultants, 2017).
2.4 Economic evaluation
To determine the economic viability of each management process and, subsequently, the feasibility of each alternative, a study was carried out in which the cost of each treatment per kg of treated waste and the cost of each alternative was calculated, considering the type of treatment and the percentage of each fraction of waste within the total generated CDW. The economic data were obtained from the construction companies, recycling plants and entities visited in Ibagué.
3. Results and discussion
3.1 Results of the management processes
The results of the management processes that are currently applied in Ibagué for each waste fraction are presented below. These results are given per kg of waste.
3.1.1 Stone recycling
On a relative scale of 0%-100%, where positive percentages represent impacts and negative percentages represent savings, Figure 4 (a) shows that the avoided process of gravel extraction contributed to the greatest savings in all the categories that were evaluated (-100%). The other processes contributed to environmental impacts. Diesel consumption had the greatest environmental impacts in all categories, apart from photochemical oxidation. The crushing process had major impacts on the category of respiratory effects (52%) due to the emission of particles. The transport process affected the soil occupation category (28%) as did the infrastructure of the recycling plant (14%). The environmental burdens of the rest of the processes were minimal.
3.1.2 Excavated earth reuse
Figure 4 (b) shows that the avoided process of earth landfilling contributed to great environmental savings in all the categories (-100%). The other processes contributed to environmental impacts, but in a smaller proportion (less than 20%). Transportation was the process that contributed most to the impacts on the categories, particularly global warming (17%), mineral extraction (13%) and photochemical oxidation (11%). Diesel consumption and leachate emissions during land compaction did not have significant impacts in the process of earth reuse.
3.1.3 Excavated earth and stone landfilling
The process of earth and/or stone disposal does not avoid any processes, which is why it contributed to positive environmental loads. The process that contributed most to environmental impacts was the infrastructure of the landfill, especially in the categories carcinogenic (81%), acidification (77%), nonrenewable energy (77%), depletion of the ozone layer (77%) and eutrophication (74%). Another process that contributed to environmental impacts was transport, particularly in the global warming category (44%). Transformation and use of earth for landfill had an impact on the land occupation category (41%) (Figure 4 [c]). Leachate emissions are not relevant in the process of earth and stone disposal, as they are inert materials.
3.1.4 Metal (steel) recovery
Metal recovery plays an important role as it avoids the process of extracting iron ore. This had environmental benefits in all the impact categories. The greatest savings were found in the inorganic respiratory (-100%), photochemical oxidation (-100%) and mineral extraction (-100%) categories. Significant savings were also generated in the following categories: acidification (-80%), reduction of the ozone layer (-37%), non-renewable energy (-29%) and global warming (-27%). The infrastructure of the classification plant contributes to greater impacts in the evaluated categories. The greatest impacts were identified in the categories of eutrophication (98%), land occupation (97%) and carcinogenic effects (91%) (Figure 4 [d]).
3.2 Comparison of the evaluated processes
In Figure 5, the processes that were evaluated are compared to determine the environmental impacts of each one per kg of waste.
Figure 5 shows that the metal recovery process contributed to impacts on the carcinogenic effects (100%), ozone depletion (67%), land occupation (100%), acidification (64%), eutrophication (100%), global warming (100%) and non-renewable energy categories (100%). However, there were also savings in the inorganic respiratory (-100%), photochemical oxidation (-63%) and mineral extraction (-100%) categories. These savings were due to avoidance of the processes of extracting and producing raw material. The results agree with those found by Mercante et al. (2012).
The stone recycling process led to savings in all categories. The biggest savings were in photochemical oxidation and acidification (-100%). This is mainly due to the avoidance of natural aggregate extraction loads. The lowest savings in the inorganic respiratory category (-1%) were due to the emission of particulate material during the stone crushing process.
As in Mercante et al. (2012), it was found that stone recycling led to savings in all categories.
The results agree with those of Mercante et al. (2012), Coelho and de Brito (2012), Kucukvar et al. (2014) ,Guignot et al. (2015) and Wang et al. (2018), who also found that recycling CDW led to environmental savings.
The process of earth reuse resulted in environmental savings in all categories, as the high loads in the earth landfilling process are avoided. The biggest savings were in the categories of ozone depletion (-89%) and non-renewable energy (-86%). Significant savings were also identified in photochemical oxidation (-46%), acidification (-38%) and soil occupation (-38%) categories.
The disposal of earth and/or stones had an impact on all categories. The greatest impacts were found in the category of ozone depletion (100%) and non-renewable energy (97%) as a result of energy consumption during the process. There were also impacts on land occupation due to the transformation and use of the earth. The impacts of leachate were not relevant in this process; therefore, eutrophication effects were not very high (4%). The results coincide with Butera (2015), who found impacts due to waste disposal and no savings from this process.
3.3 Analysis of the different alternatives
Finally, the environmental impacts of the current management system were evaluated, considering the waste fraction and the management process applied to each type of waste that make up 1 kg of the CDW generated in Ibagué (Colombia). This management alternative (A1) was compared with two other alternatives in which the percentage of the waste fraction and/or the management process was modified (A2 and A3).
The results in Figure 6 show that the current management alternative (A1) for 1 kg of CDW generated in Ibagué had an impact on all categories, except mineral extraction, in which environmental savings were found (-21%) due to the metal recovery process. The greatest impacts were in the eutrophication category (47%). This may be due to the fact that in this alternative, 100% of the stone and 50% of the excavated earth were dumped, which has a greater impact on this category due to the infrastructure of the landfill and transport of waste to the landfill. Other impacts associated with this alternative were in the categories of global warming (34%), non-renewable energy (30%), land occupation (30%) and ozone depletion (29%). These impacts are due to fuel consumption and the use and transformation of the earth in the waste disposal process. In addition, Borghi et al. (2018) evaluated the environmental performance of the regional management of construction and demolition waste in Italy. The results showed that the induced environmental impacts are generally greater than the benefits arising from recycling activities.
Alternative 2 had lower environmental impacts than A1 in all categories. In the categories of photochemical oxidation and acidification, it led to environmental savings, unlike A1, because it includes the process of recycling 50% of the stone waste, thus reducing the disposal of this material.
Alternative 3 led to environmental savings in all the categories (-100%). This is due to the fact that disposal of waste is eliminated and the percentage of stone waste recycling and reuse of earth increased. In other words, in A3 all generated CDW is recovered. Since the impacts that are avoided when waste materials are recovered and used instead of virgin material are much greater than the impacts that are generated, the net result is that recovery or recycling contributes to savings. These results are consistent with those obtained by Coelho and De Brito (2012) who found better results for management alternatives in which 96% recycling was carried out, resulting in lower environmental impacts in the categories of climate change, acidification, summer smog, nitrification and heavy metals than the alternatives in which recycling was null or scarce. In addition, Rosado et al. (2019) evaluated the environmental performance of construction and demolition waste management in Brazil. The results highlighted the importance of the impacts that are avoided by recovering materials, particularly those related to steel, glass and plastics recycling.
Table 8 shows the environmental impacts of the alternatives evaluated per kg of CDW. These results coincide with those found by Coelho and de Brito (2013a) who in their study concluded that the impacts prevented through material recycling can be up to one order of magnitude greater than those generated.
According to the World Bank (2013) Ibagué is classified as the second city with the most growth and strongest development perspective in Colombia. This is why it is believed that this region could have good demand for this type of materials for infrastructure construction.
3.4 Sensitivity analysis
It was considered necessary to carry out a sensitivity analysis to determine how the final results varied. It was assessed how the results changed when the distance to the recycling plant was altered. Previous studies were taken into account to select the distances. Rodríguez et al. (2015) indicated that the maximum viable distance between the sources of residues and recycling facilities is 30 km. Ulubeyli et al. (2017) proposed that the maximum limit for viability is 50 km between facilities. Therefore, a sensitivity analysis was required, for which scenario 3 was taken as a basis and the environmental impacts were evaluated according to the distances of 30 km (A4) and 50 km (A5).
Figure 7 reveals that A3 continued to be the most favorable scenario, due to the greater savings in the evaluated categories. Also when Coelho and de Brito (2013b) performed a sensitivity analysis, they found that in spite of the variations in overall primary energy and CO2eq balances, the prevented impacts are always higher than the generated impacts.
When the transport distance to the recycling plant was increased, savings were still made in the alternatives. The savings decreased as the distance to the recycling plant increased. These results agree with those found by Butera (2015), who considered that the process of transporting CDW to the recycling plant contributes to environmental impacts. Butera (2015) concluded that transportation contributed 30 per cent of carcinogenic human toxicity impacts, but only played a minor role with respect to non-carcinogenic human toxicity. Blengini and Garbarino (2010) reached a similar conclusion. In their case, it was concluded that the transportation distance of recycled aggregate should increase two to three times before the induced impacts outweighs the avoided impacts.
3.5 Economic evaluation
The economic factor was also relevant when different alternatives were assessed. In this study, the economic evaluation was undertaken on the basis of the cost of each management process per ton, and considering the cost avoided by the management process (Table 9).
Table 9 Economic cost of each process per kg

Cra= cost of recycled aggregate; Tc= transport cost; Clr= cost of landfill rate; Lc= labour cost (separation); Ccc= Classification and compaction cost; Cna= cost of natural aggregate; Cg=cost of earth; Sc= scrap cost
The net cost of each alternative (Table 10) was obtained from: the values of the net cost of Table 9 and the percentages of each process within the alternative (Figure 2).
Table 9 shows that A3 was the most beneficial scenario, from the economic perspective, and that increasing the distance to which the recycling plant would be located (50 km, A5) would continue to lead to economic savings, compared to the current management alternative (A1).
4. Conclusion
In this study, management processes for construction and demolition waste fractions in Ibagué (Colombia) were evaluated. The environmental impacts of the management of 1 kg of generated CDW were calculated, considering the current management of each fraction of waste and the percentage of the fraction within the total CDW that is generated (A1). New management alternatives were evaluated (A2 and A3) in which the percentage of the waste fraction or the type of treatment applied to each one was varied, to determine viability. The environmental evaluation was performed using LCA methodology. An economic evaluation of alternatives was also carried out to determine viability.
The comparison of processes evaluated per kg of waste fraction revealed that metal recovery contributed to impacts in some categories and savings in others. Likewise, the process of earth reuse resulted in environmental savings in all categories, due to the high loads of the process that was avoided (disposal of earth in landfill). The last process to be evaluated corresponded to earth and stone landfilling, which had an impact on all categories.
The comparison of management alternatives evaluated per kg of CDW revealed that A1, which corresponds to the current management of CDW in Ibagué, contributed to environmental impacts in all categories, except mineral extraction. A2 led to savings in photochemical oxidation, acidification and mineral extraction. A3 brought about great savings in all categories.
Thus, the results of this study allow us to verify that the most beneficial CDW management scenario from the environmental and economic point of view in Ibagué is that in which 100% of the metals are recovered, 100% of the earth is reused, and 100% of the stone waste is recycled.
The results could help to raise awareness among all the agents involved in the construction sector, who can clearly see the vital role they play in the proper management of CDW and, as a result, promote the implementation of mechanisms and infrastructure for waste recovery and recycling.
Likewise, the results could contribute to enhancing environmental awareness in the educational and professional field, leading to more sustainable production and consumption habits in Colombia. Finally, it is hoped to help solve current problems related to the inadequate management of CDW, and contribute to progress in this area, as has occurred at international level.