1. Introduction
The necessary use and management of lands, which are an essential part of the terrestrial ecosystem and agricultural production, without losing their functions, is only achievable by understanding all the properties of soils for gaining character. The chemical content of soils depends on the nature of the bedrock from which the soil is formed, decomposition-weathering processes, biological events, and additional materials from various sources. Accordingly, the type of the bedrock can significantly affect soil formation and the availability of nutrients. The conditions in which the bedrock is found in soils' formation cause variations in properties by affecting the weathering rates. As a result of these events, changes in physical, chemical, and mineralogical properties and different horizon structures occur (Karacan et al., 2016). Differences in soil age or bedrock can be evaluated to show the relative change in soil formation degrees where other soil forming factors are similar. In cases where soil formation factors are identical on different bedrocks, determination of chemical weathering and, consequently, clay mineral formations will enable a more successful analysis of environmental issues, and a better understanding of the cycle of elements, losses, and retention of nutrients.
As it is well known that the soil is formed as a result of different processes of the bedrock. Moreover, mineral weathering, crucial part of the pedogenetic evaluation, also supplies an essential role by transforming bedrock to weathered rock and consequently to soil that supply nutrients to terrestrial ecosystems (Tunçay et al., 2020). Soil formation is also a prolonged process. Over time and under the influence of climate, the bedrock's impact on soil mineralogy and physico-chemical properties is thought to decrease, but the time required is uncertain (Wilson et al., 2017). The changes in the soil's clay mineralogy due to the bedrock differences have also been demonstrated by previous studies (Benedetti et al., 2011; Heckman & Rasmussen, 2011). In a study conducted by Benedetti et al. (2011), it was concluded that high acidity and low Fe oxide content were dominant in the kaolinite-bearing sediments with low productivity levels, while the presence of high base saturation and 2: 1 type clay minerals were identified in areas with basalt and alluvial effects. Şenol et al. (2020) stated clay accumulation in the low slope position lands due to topography, and weak vegetation and sand content in soils was located on volcanic rocks such as tuff and andesite. Micro-elements and macro-elements were also detected at low levels in soils formed on the same tuff bedrock. Fiantis et al. (2010) in the study on the determination of trace element contents and geochemical fragmentation weathering indices in volcanic ash deposits in Indonesia; It has been reported that K, Ca, and Na originate from feldspar, pyroxene and hornblende, and sulfate anions formed as a result of high S content in volcanic ash are held to the A and B horizons. They explained that olivine, hornblende, and augite in volcanic ash caused the increase in Ni, Zn, V, and Cu contents in the profile. In a study in which they tried to determine the effects of organic matter on soil and nutrient content in soils with varying degrees of fragmentation and decomposition. Hauser & Billing (2017) discovered that in profiles with high chemical alteration index (CIA) and high decomposition degree, organic matter decomposes faster than other profiles.
Previous studies clearly indicate that in addition to the bedrock, climatic conditions are an important factor in weathering since climate determines the weathering product of volcanic materials and therefore the type of noncrystalline minerals that will be formed (Arikan et al., 2007; Gündoğan et al., 2009; Gündoğan et al., 2012; Özpinar, 2008; Kibici et al., 2012; Uzun, 2013; Şenol et al, 2014; Sönmez &Ku§cu, 2020; Şenol er al., 2020). However, there are not enough studies on rocks of volcanic origin in Turkey and in the study area. In addition, revealing the difference in volcanic rocks under the same climatic conditions separates the study from previous studies. The present study aimed to compare young soils developed on different type of rocks by: i-) the determination of essential geo-physico-chemical characteristics and a morphological description; ii-) the properties of the primary and secondary minerals and iii-) the changes in fragmentation-weathering indices of the relationship between bedrock and soils formed on different volcanic rock types and defined as young soils, under the influence of the same land use-land cover and climatic conditions within the borders of Sandikli district of Afyonkarahisar province.
2. Material and method
2.1 General Features of the Field of Study
The study area covers approximately 295 km2 between Sandikli, Kizilören, and Şuhut districts within the provincial borders of Afyonkarahisar in Central Anatolia of Turkey. It is among the map sheets of K24-c3, K24-c4, L24-a2, L24-b1, L24-b2, L24a3, and L24-b4 scaled as 1: 25.000 and 251000-286000E-4232000-4272000N coordinates (WGS84, Zone 36, UTM m) (Figure 1). Sandikli district and its surroundings have a minimum elevation of 1064 m, a maximum of 2121, and an average of 1424 m. The elevation increases in the east and northeast parts of the field of study. Depending on the rise in altitude, topographic features change as hilly and mountainous positions, and the slope increases.
According to Corine (2018), the land use types of the study area are 35.21% agricultural land (10406.93 ha), 64.10% forest and semi-natural areas (18942.27 ha), and 0.69% artificial areas. Wetlands and water bodies cover 5.49% of the area. The area considered for the three soil profiles (PI, PII, and PIII) formed on different volcanic bedrocks has been adopted as pastureland for many years (more than 50 years). According to the Old American Soil Classification of the study area (Baldwin et al., 1938), 42% is "Non-Calcareous Brown Soils," 16% "Chestnut Soils," 7% "Colluvial Soils," 5% "Brown Soils," 29% is "Bare Rocks and Rubble" and the remaining part is "Alluvial Soils". According to the study area's long-term climate data (Afyonkarahisar-Şuhut Meteorology station observation data), the annual average temperature is 10.8 oC, precipitation 362.40 mm, and evaporation 663.08 mm. The highest rainfall in the region is in December (43.9 mm) and the least in September (11.7 mm). Besides, Newhall Simulation Model was applied to determine the soil moisture and temperature regimes of the area (Newhall & Berdanier, 1994; Van Wambeke, 2000). According to the result, the soil temperature regime was determined as "Mesic" and the soil moisture regime as "Xeric" (Dry Xeric" as the subgroup) (Figure 2).
As a geological pattern, there are metamorphic, sedimentary, and volcanic units in around the Sandikli district, including the study area. Metamorphics are composed of schist and quartzites, sedimentary units, dolomitic limestone, limestone, pebblestone, sandstone, siltstone, claystone, clayey limestone, neritic limestone, alluvial fan deposits, and alluviums. Volcanic units cover volcanoclastic rocks (Tuff, Agglomerate) and volcanic lavas (Trachyte, Trachyandesite, Trachybasalt, Basaltic trachyandesite).
2.2 Soil and Rock Sampling
Within the study area, the geological units where the profile points are located were determined from the geology map (MTA, 2011), and ground controls and validation were made. Three representative profiles were excavated in the determined areas. In the geology map of the study area, it was determined that a large part of it was formed by volcanic rocks. Considering this situation, the fact that most of the profile points determined in the study area are volcanic rocks has increased the representativeness of the study area. Each profile was made morphological definitions, and profile description was made according to Soil Survey Staff (1993), and genetic soil horizons were symbolized according to Soil survey staff (1999). In addition, rock samples were taken from R horizons that show the bedrocks of the profiles (Figure 3). Soil samples were taken disturbed and undisturbed based on the horizon (Soil Survey Staff., 2014). Soil and rock samples were brought to the laboratory.
2.3 Soil Analysis
Descriptive physical and chemical analyses in soils were made on 2 mm and 0.5 mm sieved soil samples. Particle size distribution was determined by the hydrometer method according to Bouyoucus, (1951), and soil color in dry and moist conditions using the Munsell color scale according to Soil survey staff, (1993). Soil pH and EC and salt concentration in saturation mud according to Richards, (2012), lime contents with Scheibler calcimeter, the volumetric method according to Kacar, (2009), and organic matter with modified Walkley-Black method according to Jackson, (1958). Exchangeable cations (Mg, Ca, Na, and K), free Fe2O3, and free Al2O3 were determined according to Perkin Elmer, (1973). The number of available micronutrients (Fe, Cu, Zn, and Mn) was determined by the DTPA method proposed by Lindsay & Norvell, (1978) (Kacar, 2009). For a total analysis, dried ground soil and rock samples (O Φ38 µn) were extracted according to Chao & Sanzolone, (1992) and made ready for analysis. After the extraction process of major elements (Si, Al, Ca, Mg, Na, K, Ti, and P) and trace elements (Fe, Cu, Zn, and Mn) are determined in the ICP-OES device.
The decomposition weathering indices of the soils were determined according to the formulas given below.
Chemical Alteration Index (CIA)= (100) [Al2O3/ (Al2O3+CaO+Na2O+K2O)] (Nesbitt and Young, 1982)
Chemical Weathering Index (CIW) = (100) [Al2O3/(Al2O3+CaO+Na2O)] (Harnois, 1988)
Plagioclase Alteration Index (PIA) = (100) (Al2O3- K2O)/ (Al2O3+CaO+Na2O+K2O) (Fedo et al., 1995)
Productivity Index (P) = (100) [SiO2/(TiO2+ Fe2O3+Al2O3+SiO2) (Reiche, 1950)
Bases/R2O3 = (MgO+ CaO+Na2O+K2O)/(TiO2+Fe2O3+Al2O3) (Birkeland, 1999)
2.4 Determination of Primary Minerals
The proportional distribution of primary minerals in soils was determined in samples ground with a Bruker D8 Advance X-ray diffractometer (in the range of 2 θ =2-70o) (Jackson, 1958). The abundance degrees of the primary minerals were revealed in the shootings. Washing, decantation, lime removal, centrifugation, and sedimentation were carried out to determine the clay mineral type in the ground samples. The resulting clay fractions were separately saturated with 1N MgCl, and KCl washed and plated. Purified clay samples were placed on a slide and oriented with ultrasonic vibrations for about 15 minutes. Referred examples separately; XRD analyzes were performed on each preparation prepared by 1) air drying, 2) treatment with ethylene glycol for 2 hours in an oven at 60 °C, and 3) conditioning at 550 °C for 2 hours. Shootings were performed on the XRD device (in the range of 2θ=2-15o) (Whittig & Allardice, 1986). Thin sections of rock samples were made, and photographs of the rocks' minerals and textures were taken under the microscope (Murphy et al., 1977).
3 Results and discussion
3.1 Morphological and Physico-Chemical Properties of Soils
Morphological definitions of profiles selected in the study area are given in Table 1. Analysis results of physico-chemical properties are also shown in Table 2. Profiles were classified according to the soil taxonomy, considering surface (epipedon) and subsurface diagnostic horizons. It was determined morphological, mineralogical, and physico-chemical differences in the three soils formed on the volcanic bedrocks under the same climatic conditions. These soils are young soils. Soils have wide ranges of slope (6-12%) and have an A/R horizon sequence, and in the profiles do not contain a subsurface diagnostic horizon and advance pedological process. These three representative profiles were classified as Lithic Xerothent (Soil Survey Staff, 2014). On the other hand, soils formed on tuff (PI) and Trachyandesite (PIII) is classified as Lithic Leptosol, whereas soil formed on Trachybasalt (PII) was classified as Mollic Leptosol according to FAO / ISRIC, (2014). Due to the high levels of clay and organic matter content, the soil structure formed on Trachybasalt exhibits a strong, medium granular, and semi-angular block structure. Simultaneously, in other surface horizons of profiles, it has been determined as a small, weak granular structure. The darkest color in the profiles' surface horizons was determined with 10 YR 3/1 in the soil formed on Trachybasalt.
Table 1 Morphological properties and classification (Soil Taxonomy (Soil Survey Staff, 2014)-FAO/ISRIC (2014) of profiles.
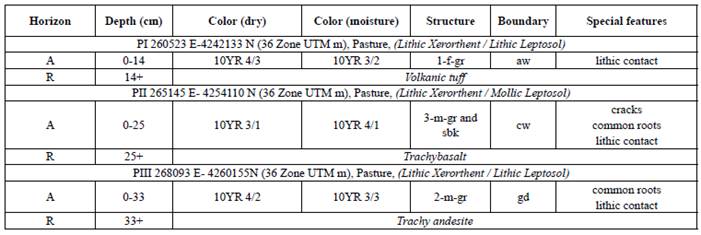
Abbreviations: Boundary: a = abrupt; c = clear; g = gradual; d = diffuse; s = smooth; w = wavy; i = irregular. Structure: 1 = weak; 2 = moderate; 3= strong; sg = single grain; mas = massive; vf = very fine; f = fine; m =medium; c = coarse; gr = granular; pr = prismatic; abk = angular blocky; sbk = subangular blocky.
Table 2 Some physical and chemical analysis results of profiles
PI (Tuff) | PII(Trachybasalt) | PIII (Trachyandesite) | |
---|---|---|---|
pH | 7.76 | 7.78 | 6.93 |
EC (dS/m) | 0.14 | 0.15 | 0.08 |
Clay (%) | 22.23 | 26.27 | 15.93 |
Silt (%) | 35.15 | 25.93 | 18.36 |
Sand (%) | 42.62 | 47.80 | 65.71 |
CaCO3 (%) | 12.43 | 1.23 | 0.99 |
Na (cmol(+) kg-1) | 0.42 | 0.12 | 0.06 |
K (cmol(+) kg-1) | 13.00 | 0.63 | 0.45 |
Ca (cmol(+) kg -1) | 39.87 | 37.43 | 5.39 |
Mg (cmol(+) kg-1) | 1.55 | 4.39 | 1.13 |
Cu (mg kg-1) | 2.22 | 1.59 | 1.09 |
Mn (mg kg-1) | 16.82 | 38.20 | 28.58 |
Fe (mg kg-1) | 6.75 | 23.39 | 11.09 |
Zn (mg kg-1) | 0.65 | 0.98 | 0.76 |
P (mg kg-1) | 14.80 | 6.72 | 10.60 |
OM (%) | 2.37 | 2.80 | 1.72 |
Soils, according to Dhanke &Whitney (1988), are slightly acid-slightly alkaline reactions and are in the salt-free class according to their EC content. While the lowest pH value and salt content were found in Lithic Xerorthent shown with the PIII (Profile 3) profile formed on the Trachyandesite bedrock, the highest values of them were determined in the soils located on Trachybasalt bedrock, which was coded as PI. The high level of basic cations in PI (Profile 1) and PII (Profile 2) is considered a reason for increased pH levels. The dominant cation in all three soils is calcium ion.
However, while it is mostly found in the soil formed on tuff (39.87 cmol kg-1), the lowest (5.39 cmol kg-1) was determined in the soil located on Trachyandesite. In addition, it has been concluded that zeolite, feldspar, and albite feldispare dominate the primary mineral definitions in the soils formed on the tuff-one of the reasons for the high levels of cations in the presence of these minerals. Primary minerals are included Calcite, Sanidine, Microcline, Quartz, Muscovite, Albite, Gismondin, Phillipsite, Orthoclase, Ankerit, Chabasite, and Anorthoclase. In this profile, calcite mineral is abundant. Accordingly, high levels of Ca cation are expected. The abundance of calcite minerals supports that the calcium (mg kg-1) amount for the PI profile is higher than that of the PII and PIII profiles (Table 2). Besides, smectite minerals were determined in PI and PII. The fact that precipitation is not enough to cause basic ions to leach in the soil profile significantly affects the soil's clay mineral type. Smectite is formed when the cations are not leached from the soil at a sufficient level (Uzun, 2013).
The soils' calcium carbonate content is very variable. While it is 12.43% in soils on tuff, it is relatively low in other soils. In fact, soils formed on volcanic materials, especially tuff, basalt, or trachyandesite, do not contain calcium carbonate. Tunçay & Dengiz, (2020) explained that high calcium carbonate content in the profiles they work with could be caused by the i-) contamination of volcanic materials by calcareous materials during lava flows, ii-) that calcareous materials may be transported by winds and contaminated volcanic materials, or iii-) recrystallization of hydrothermal waters rich in calcium carbonate within porous lava and basalt materials. Similar to our findings, Şenol et al., (2018) determined the lime content between 0.09% and 6.37% in their studies on geochemical mass index and clay mineral formation of soils formed on the primary basalt material and in different topographic positions under semi-humid climatic conditions. As the constituent classes of the working soils, the trachyandesite material's soils are more coarse and sandy loam. The texture of the bedrock and the land's topography are the parameters that affect the change of soil properties (Osher & Buol 1998). Krasilnikov et al., (2005) reported that texture and mineralogical structure are factors in the relationship between geomorphology and formation in soils. It is stated in the literature that soils developed on tuff bedrock have high lime content. This situation shows the transport and accumulation of different rocks due to tillage and topography.
It results from the precipitation of limestone dissolved by precipitation on the soils formed on the tuff.
Although all three soils are covered with pasture vegetation, when the soils' organic matter contents were examined, the soils on Trachybasalt were higher. Basic cations were detected at high levels in soils over Tuff and Trachybasalt, respectively. The PI profile contains anorthoclase mineral higher than, unlike PII and PIII. This mineral is known as a mineral group rich in cations. Besides, due to minerals such as pyroxene and olivine in these soils, the microelement and especially iron content were determined to be higher than in other soils (PII and PIII). The high iron content in the PI profile is associated with the low amount of aluminium oxide which is the chemical composition Al2O3. Since the amount of iron oxide forms more stable compounds with the increase in aluminium oxide, it supports the lower iron content in the PII and PIII profiles than the PI profile.
3.2 Mineralogical andPetrographic Investigations of Rocks
Thin section images of the bedrock on which the soils are formed are given in Figure 4. The PI profile's bedrock is tuff; a highly hollow, soft, and brown-colored pyroclastic rock with volcanic rock and mineral fragments in an ash matrix. Rock fragments are generally composed of volcanic rocks such as trachyte, trachyandesite, and basaltic trachyandesite. In contrast, minerals are represented of crystals such as sanidine, plagioclase, pyroxene, and mica. Sanidines are subhedral and sometimes euhedral and have a clean appearance. Likewise, Kibici et al., (2012) mentioned the presence of very fine-grained sanidine crystals in their study to reveal the mineralogical and petrographic characteristics of the volcanic rocks of the Afyonkarahisar region.
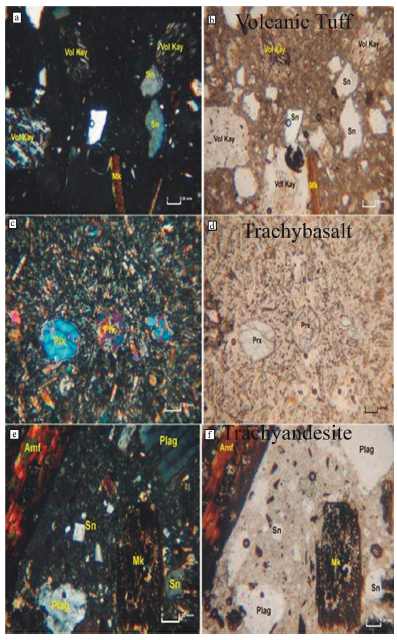
Figure 4 a, b: Microscopic images of tuff, which is a pyroclastic rock. Ash is composed of volcanic rock fragments in a matrix and crystals such as sanidine and mica. a) double polos (plane-polarized light) b) single pools (single polarized light); c, d; Pyroxene phenocrysts forming the Trachybasalt rock are contained in a microlithic groundmass. c) double pounds, d) single pounds. Prx: Pyroxene; e, f; Microscopic image of plagioclase, amphibole, mica phlogopite or biotite and sanidine crystals in a matrix of sanidine, plagioclase and mica microliths in combination with volcanic glass in trachyandesite rock. e) double pounds, f) single pounds.
The bedrock of Lithic Xerorthent soils, shown as PII, is Trachybasalt, a gas-filled, grayish, fine-grained, and aphanitic textured rock. The rock is composed of silica (quartz and/or tridymite), sparse mica, and apatite minerals in gas cavities, together with pyroxene phenocrysts in a paste consisting of sanidine, pyroxene, and opaque mineral microlites. Pyroxenes, which are found as phenocrysts, appear in two forms: (1) thin-long rod-like crystals and (2) short prisms. They are generally euhedral and subhedral (Figures 4c and d). Approximately 85-90% of the rock consists of microlithic paste, and the remaining part is composed of minerals found as phenocrysts.
Lithic Xerorthent, coded as PIII, is a porphyric textured volcanic Trachyandesite rock with marked sanidine and plagioclase crystals, the primary material of the soil was affected by severe alteration. The rock comprises plagioclase, sanidine, pyroxene, mica (phlogopite or biotite), and amphibole main minerals (Figure 4d, f). Apatite and opaque minerals are available as accessories. Some researchers have stated that the minerals forming the rock appear in a matrix consisting of sanidine, plagioclase, and mica microlites together with volcanic glass (Kurt, 2009; Kibici et al., 2012). Plagioclases constitute 15-20% of the main minerals, 10-15% of sanidine, 10-15% of pyroxene, 10-12% of mica, and 8-10% of amphibole. Apatite has 1-2%.
Plagioclases are seen as polysynthetic twinnings, usually clean and clear. They are euhedral and subhedral and some have zoned extinction. They are broad plates and short prism shaped. Sanidine crystals are rectangular, and some of them have rounded corners by being eroded by magma fluids. They are euhedral, subhedral, Carlsbad twinning, and slightly clayed due to a low alteration degree. Pyroxenes are short blunt and long rod-like, euhedral and subhedral. They come in octagonal, hexagonal, and sometimes rectangular shapes. They show simple twinning, are gathered together with other minerals, and form glomeroporphyric texture. Micas are generally opaque by oxidation. They are found in short and sometimes long rectangular plate-like shapes. Amphibole crystals, like micas, are opaque, especially by oxidizing at the edges. Opacification has surrounded the edges of the mineral as a belt or completely encircled it. It is hexagonal in euhedral and subhedral and has bidirectional cleavage. It shows a greenish and brown pleochroism in crossed nicols and a brown pleochroism in parallel nicols. The crystals were separated from the edges and middle sections by being corroded by the magma liquid. Rare apatites are in short blunt and pithed shapes and become clay. The rock has a porphyritic and glomeroporphyric texture.
3.3 Primary and Clay Minerals of Soils
X-ray peaks for primary minerals in the soil formed on tuff bedrock are given in Figure 5. Determination of primary minerals was carried out in the range of 2-70o (29) nontronite, muscovite, sanidine, gismondine, albite, calcite, and phillipsite minerals were determined. Calcite, albite, muscovite, gismondine, sanidine, phillipsite, and nontronite were determined in order of densities (Table 3). Albite is a mineral commonly found in schists, which forms the basis of the study area. Like the muscovite mineral albite, it is included in the composition of metamorphic rocks (schists). It can also occur secondary minerals to the transformation of the feldspars due to clay minerals decomposition. Also, gismondine [(CaAl2Si2O8 x 4(H2O)] and phillipsite [(Ca,Na2,K2)3Al6Si10O32 x 12(H2O)] which are zeolite minerals, were detected in these soils. Özpinar, (2008) stated that there are three different forms of phillipsite, potassium-sodium-aluminum-silicate hydrate, potassium-aluminum-silicate hydrate, and potassium-calcium-aluminum-silicate hydrate, in tuffs in the south of Sandikli district.
Table 3 Primary minerals and densities of soils formed on different volcanic parent rock
Profile | Horizon | a | b | c | d | e | f | g | h | i | j | k | l | m | n |
---|---|---|---|---|---|---|---|---|---|---|---|---|---|---|---|
PI | A(0-14) | +++ | ++ | - | - | ++ | + | + | + | - | - | - | - | +++ | - |
PII | A(0-25) | - | ++ | +++ | - | ++ | - | - | - | - | + | - | + | - | |
PIII | A(0-33) | - | ++++ | - | - | ++ | - | - | - | +++ | ++++ | - | - | - | - |
Abundance: ++++ Very Abundant, +++ Much Abundant, ++ Medium Abundant, + Low Abundant; Minerals: a: Calcite, b: Sanidine, c: Microcline, d: Quartz, e: Muscovite, f: Albite, g: Gismondin, h: Phillipsite, i: Orthoclase, j: Ankerit, k: Chabasite, l: Anorthoclase, m: Nontronite, n: Montmorillonite |
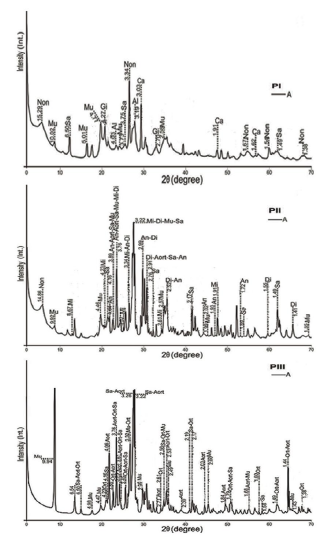
Figure 5 Soil profile X-Ray diffractions of primary minerals (Mi: Microcline, Di: Diopside, Mu: Muscovite, Sa: Sanidine, An: Anorthite, Aorta: Anorthoclase, Non: Nontronite, Ca: Calcite, Al: Albite, Gi: Gismondin, Phl: Phillipsite, Ort: Orthoclase)
X-ray diffractometers were taken to determine the mineralogical properties of soils (PII) formed on Trachybasalt are given in Figure 5. Determination of primary minerals in the soil sample was carried out in the range of 2-70o (2θ) nontronite, muscovite, anorthite, microcline, sanidine, anorthoclase, and diopside minerals were determined. In order of mineral abundance, they are microcline, diopside, muscovite, sanidine, anorthite, anorthoclase, and nontronite minerals (Table 3). Anorthoclase is a mineral formed at the high temperatures of volcanic and semi-deep rocks. It is an intermediate member of the alkali feldspar, albite-sanidine solid solution series. Anorthite (CaAl2Si2O8) came to the environment by being carried from peridotite rocks. In mineralogical and petrographic investigations, it was stated that sanidine is composed of silica (quartz and/or tridymite), rare mica, and apatite minerals found in gas cavities together with pyroxene phenocrysts in a paste consisting of pyroxene (diopside) and opaque mineral microlites. Microcline is found in rocks such as syenite, granite, syenite porphyry, and granite porphyry. Sönmez & Kusçu, (2017) stated that K-feldspar syenites were found in the Afyonkarahisar-Sandikli region. Gündoğan et al. (2009; 2012) mentioned the presence of the Karacaören syenitoid east of Sandikli (Afyonkarahisar).
An X-ray diffractometer was taken to determine the primary mineralogical properties of the PIII profile are shown in Figure 5. Muscovite, anorthoclase, and orthoclase minerals have been identified as primary minerals in the soils. Sanidine, anorthoclase, orthoclase, and muscovite were determined in order of abundance (Table 3). Similarly, in their study to reveal the mineralogical and petrographic properties of the Afyonkarahisar region's volcanic rocks, Kibici et al., (2012) mentioned mica's presence mineral and very small-grained sanidine crystals in a paste of trachyandesite rock.
X-ray peaks of clay minerals of young soils classified as Lithic Xerorthent but formed on different volcanic bedrock are given in Figure 6. In the soil sample, which is stated as PI and formed on the tuff bedrock, in the samples saturated with Mg (Mgad), the crystallized peaks were opened up to 18.39 Å in the application of MgEG (Mg Ethylene Glycol). In potassium saturation (Kad), these peaks are closed to the range of 12.87. Peaks indicate the presence of crystallized smectite minerals. The peaks seen in the range of 9.92-10.11 Å in all applications belong to crystallized illite. The peaks are seen in the 7.18-7.23 Å range of samples saturated with Mgad, MgEG, and Kad disappeared when heated at 550 oC (K550 oC). This situation indicates that these peaks belong to kaolinite. Very weakly crystallized peaks seen in the range of 11.77-12.87 Å in all applications showed that clay minerals with Illite-chlorite interlayer existed. In the order of abundance, the distribution of clays was in the form of smectite, illite, and kaolinite (Table 4). Şenol et al. (2014) identified smectite as the dominant clay mineral on south-facing slopes in a study on clay mineralogy of soils formed on trachyte / trachyandesite. Uzun, (2013) stated that the basic cations released due to the amphiboles' decomposition increase the pH and the Si, Mg, and Ca cannot be washed at a sufficient level, smectite clay mineral is formed.
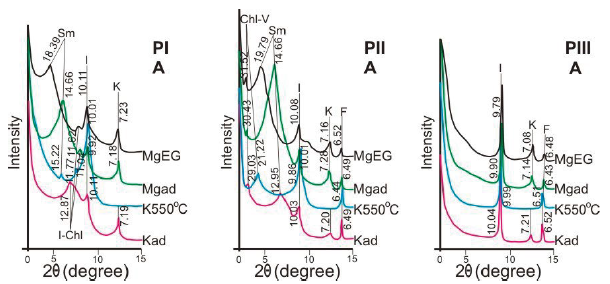
Figure 6 X-ray peaks of soils formed on different volcanic parent rock (Sm: Smectite, K: Kaolinite, I: Illite, Chl-V: Chlorite- vermiculite, F: Plagioclase felspars)
An X-ray diffractometers of clay types in the surface sample of young soil profile formed on Trachybasalt and coded as PII are given in Figure 6. The 14.66 Å crystallized peak in the samples saturated with Mg (Mgad) in the soil sample was opened up to 19.79 Å in the treatment of MgEG. This peak is closed to 12.95 Å at saturation with potassium. The peak indicates the presence of crystallized smectite minerals. The peaks seen in the range of 9.86-10.08 Å in all treatments belong to the crystallized illite. The samples saturated with Mgad, MgEG, and Kad peaks seen in the range of 7.16-7.28 Å disappeared when heated at 550oC. Therefore, these peaks were identified as belonging to kaolinite. In samples saturated with Mg in the profile, the weakly crystallized peak (MgEG) was opened to 31.52 Å in the treatment. In potassium saturation and K550oC treatments, this peak is closed to 29.03 and 21.22 Å, respectively. The peak indicates the presence of a weakly crystallized chlorite-vermiculite interbedded clay mineral. The peaks seen in the range of 6.36-6.53 Å in all treatments are feldspar minerals. In the order of abundance, the distribution of clays is in the form of clay with smectite, illite, kaolinite, and chlorite-vermiculite interlayer (Table 4).
Table 4 Clay minerals and abundance of soils formed on various volcanic parent rock
Profile | Horizon | Smectite | Illite | Kaolinite | |||
---|---|---|---|---|---|---|---|
Abundance | Crystallinity | Abundance | Crystallinity | Abundance | Crystallinity | ||
pi | A(0-14) | ++++ | ++++ | +++ | ++++ | ++ | ++++ |
pu | A (0-25) | ++++ | ++++ | +++ | +++ | ++ | ++ |
phi | A (0-33) | ++++ | ++++ | +++ | +++ | ||
Abundance: ++++ Very Abundant, +++ Much abundant, ++ Medium abundant, + Low abundant; Crystallinity: ++++ Very Good, +++ Good, ++ Fair, + Bad |
The peaks seen in the range of 9.79-10.04 Å in all soil sample treatments on trachyandesite belong to crystallized illite. The peaks seen in the 7.087.21 Å range of samples saturated with Mgad, MgEG, and Kad disappeared when heated at 550oC (Figure 6). It indicates that these peaks belong to weak crystalline kaolinite. The peaks seen in the range of6.43-6.52 Å in all treatments are feldspar minerals. The distribution of clays in the order of abundance was in the form of illite and kaolinite (Table 4). The acid or alkaline reaction of soil is directly related to the OH3+ and OH- concentrations in the soil solution. A one-unit increase in pH means a 10-fold increase in the concentration of H ions. The higher pH (H2O) values might be related to lower Al activity and lower organic matter content. The pH values (in most cases above 6.0) were relatively high considering the nature of the bedrock and the absence of carbonates. PIII profile pH value contains approximately 10-fold lower H+ ion concentration than PI and PII profiles. The pH values (in most cases above 6.0) were relatively high considering the nature of the bedrock and the absence of carbonates. 2:1 type clay formation is significant in the study area profiles. It increased the pH due to the basic cations released as a result of the decomposition of amphiboles. Smectite was formed as a result of low precipitation in the study area and insufficient leaching of Si, Mg, and Ca. The very abundance of smectite for PI and PII profiles was found to be appropriate with the results of previous studies (Uzun 2013). In the study area, the PIII profile containing plagioclase, amphibole, mica phlogopite or biotite, and sanidine is a distinguishing feature from the soils with PI and PII profiles formed on the volcanic rock in terms of the absence of 2:1 type clay. The influence of pedogenesis factors is lower for the PIII profile. Except for semi-arid ecological conditions, different processes in pedogenesis factors show that soils are effective on clay fractions (Özaytekin & Karakaplan, 2012). Cangir & Ekinci (1991) identified the dominant clay mineral as illite in shallow profiles formed on the calcareous base material. The dominant clay mineral in this soil profile is smectite. On the other hand, clay mineral with chlorite-vermiculite interlayer was found in the soils located on Trachybasalt. Ince, (1979) identified smectite as the dominant mineral in the clay fraction in the soils formed on the basalt rocks in and around Diyarbakir, followed by kaolinite, chlorite, and illite.
3.4 Weathering Index
Many different indices have been used in defining the decomposition-weathering degree of soils. Chemical weathering indices are commonly used to characterize weathering profiles, typically calculated from molecular ratios based on the assumption that aluminium is immobile. This assumption is not always warranted. In addition, the molecular ratio of each oxide is easily calculated from the percent of the oxide based on weight (Nesbit & Young, 1982; Harnois, 1988; Dengiz et al. 2013). Dengiz et al., (2013) stated that weathering indices for weathering profile samples are conventionally estimated using the molecular proportions of major element oxides. In addition, the determined indexes are generally determined to reveal the exchange rates between basic cations and cations such as Al and Si. The CIA index reflects the ratio of primary and secondary minerals as the rate of removal of basic cations from minerals by chemical weathering. In the case of severe weathering or decomposition, the CIA value can be determined up to 100 levels. This index, which reflects the alteration degree of feldspars into clays after hydrolytic decomposition, may decrease to 50% in the initial stage of 100% decomposition in clays with intense decomposition (Fedo et al., 1995; Şenol et al., 2014). Nesbit and Young (1982) classified the CIA values (%) as poorly weathered (50-60), low weathered (6080), highly weathered (80-90), and excessive weathered (90-100).
Soils with the symbol PI, PII, and PIII were classified as Lithic Xereorthent according to soil taxonomy. Some weathering rates of these pedons and some major and minor elements obtained from their geochemical properties are shown in Table 5. According to the soils' CIA values, the PI soil profile formed on the tuff was determined as "slightly weathered". In contrast, the PII and PIII soil profiles were defined as "unweather" (≤ 50). CIW values increase to 50% in rocks that have not undergone disintegration. This index rises to 100% depending on the degree of growing fragmentation disintegration. Since the CIA classification is valid within the CIW, the PI was determined as "highly differentiated (89.81%)". Other profiles were found to be free from fragmentation and decomposition according to CIW values. Weathering degrees in the primary rocks of the soils are determined at deficient levels.
Table 5 Weathering index and major elements content of soils
PI (Tuff) | PII (Trachybasalt) | PIII (Trachyandesite) | ||||
---|---|---|---|---|---|---|
A | R | A | R | A | R | |
CIA | 77.04 | 42.27 | 22.88 | 0.03 | 31.40 | 0.21 |
CIW | 89.81 | 48.90 | 24.43 | 0.03 | 36.88 | 0.21 |
PIA | 62.81 | 28.72 | 16.53 | 0.01 | 16.56 | 0.21 |
Product Index | 83.31 | 87.26 | 80.89 | 99.09 | 82.71 | 88.8 |
Base/R2O3 | 0.19 | 1.18 | 2.49 | 1.09 | 1.83 | 0.99 |
SiO2 | 54.12 | 57.93 | 51.97 | 65.34 | 57.33 | 69.76 |
Al2O3 | 9.77 | 11.32 | 13.52 | 10.44 | 17.84 | 9.57 |
Fe2O3 | 9.54 | 3.39 | 6.22 | 4.68 | 5.18 | 3.7 |
CaO | 0.54 | 4.25 | 7.91 | 5.57 | 4.29 | 3.13 |
MgO | 0.24 | 0.6 | 3.42 | 2.16 | 2.37 | 0.79 |
Na2O | 0.08 | 2.49 | 2.11 | 3.14 | 3.7 | 3.31 |
K2O | 1.66 | 3.34 | 3.04 | 6.82 | 5.84 | 6.94 |
TiO2 | 2.03 | 0.7 | 1.16 | 1.03 | 1.16 | 1.01 |
P2O5 | 0.08 | 0.19 | 0.34 | 0.53 | 0.44 | 0.54 |
MnO | 0.26 | 0.08 | 0.14 | 0.09 | 0.11 | 0.11 |
CIA: Chemical Index of Alteration, CIW: Chemical Index of Weathering, PIA: Plagioclase Index of Alteration
Plagioclase Alteration Index (PIA) is used to reveal the degree of alteration of plagioclases. Again, as in other indices, the highest PI number was determined in the profile. PIA index varied between 0.01-62.81%. Although such high results in all profiles indicate that the weathering is advanced, these values can be increased on young volcanic rocks (Sayyed & Hundekari, 2006). If there is an increase in MgO, CaO, Na2O and K2O elements depending on the depth in profiles, CIA, CIW, and PIA indices tend to increase with depth, Al2O3 values decrease as a result of clay movement (Alsalam et al., 2020). CIA, CIW and PI parameters were determined in the lowest PII (trachybasalt) and the highest in the PI profile. A similar situation is valid for the product index (P). With this ratio, the values should tend to decrease due to the increase of decomposition and weathering. The P index was determined between 80.8999.09%. It is reported that such high values may occur on young rocks (Sayyed & Hundekari, 2006). The base/R2O3 ratio varies between 0-10 in soils (Şenol et al., 2014). It gives high values in the bedrock. In the PI profile, the index value decreases with depth. The increase in index with depth between the A horizon and the bedrock indicates that the PI profile is at an advanced level of disintegration. The fact that the Bases/R2O3 ratio, which gives results between 1-10 under normal conditions, is below low in the profiles (except the A horizon of the PI profile) is a result of the basic cations not being leached sufficiently and the SiO2 molar ratios at high levels due to the transport from the bedrocks. SiO2 values of the soils were determined between 51.97-69.76%. The amount of Al2O3 in soil profiles showed results close to the primary material values to support less intense decomposition. Alkali cation values in profiles differ from the bedrock. While the increase of alkali cations in the surface horizon indicates the vegetative cycle, the low level indicates a little washing in the profile. Cao, MgO, Na2O, K2O, and P2O levels were determined in the lowest PI (Tuff) profile. MgO values ranged from 0.24-3.42%. MgO is very sensitive to leaching process (Alsalam et al., 2020; Arikan et al., 2007; Nordt & Driese 2010; Regassa et al., 2014). Total TiO2 in profiles showed a distribution between 0.70-2.03%. The % amounts of TiO2 which is resistant to decomposition, reveal the decomposition level of the soil. P2O5 of soils showed distribution between 0.08-0.54% and MnO between 0.08-0.26%.
4 Conclusion
In this present study, the pedological developments, taxonomic classifications, primary minerals, and clay mineralogy and their weathering indices of young soils formed under the same climate and land cover but on different volcanic rocks in the area between Sandikli-Şuhut districts of Afyonkarahisar province with semi-arid ecological conditions were studied. Their pedological developments were examined and compared. Therefore, three soil profiles formed on the bedrock of tuff, trachybasalt, and trachyandesite from volcanic rocks in the study area were analyzed. All profiles have a medium slope, under pasture cover, in A/R horizon order. It is classified as Lithic Xerorthent due to the absence of subsurface diagnostic genetic horizon and lithic contact within 50 cm depth. The existence of sanidine and muscovite primary minerals for all profiles is in question. Differences in primary minerals have been discovered depending on the difference in volcanic units. Smectite, illite, and kaolinite clay minerals were found in soils formed on the tuff (PI) and trachybasalt (PII) primary materials. Illite and kaolinite clay minerals were discovered in trachyandesites (PIII). In the surface soils formed on tuff, fragmentation and weathering were determined at higher levels than other soil profiles (PII and PIII). In addition, significant changes in some physicochemical properties of soils were determined depending on the main bedrock differences. Zeolite and calcite minerals are found at high levels in the soils formed on the tuff bedrock where basic cations are high.
With this current study, it has been revealed that under the same climate, land cover, and slope conditions, there are significant changes in the disintegration-weathering processes and the soils' mineralogical and physico-chemical properties of volcanic bedrock. It was concluded that changes in the degree of disintegration under the same climate, land cover, and slope conditions can be successfully quantified through CIA, CIW, PIA, P, bases/ R2O3, mass transport function, and mass loss/gain, and clay mineralogy can be used to determine the degree of disintegration at short distances. Consequently, these soils are still under the bedrock's active influence at the beginning of the formation process. Besides, it is suggested that the physicochemical properties and the mineralogical properties should be determined to make the necessary studies such as soil survey mapping to be used in future studies for the sustainability of the soil by revealing these properties.