INTRODUCTION
The cultivation of tomato (Lycopersicon esculentum Mill.) is notable for its high world consumption, whether raw or processed, with great socioeconomic importance (Ferreira et al., 2017). Because it is a highly profitable activity with wide acceptance in the consumer market, the tomato is the most commercially important vegetable crop in Brazil, with an average production of 4 million tons of fruits harvested annually, in an area of approximately 60,000 ha (IBGE, 2016).
Of the several biotic and abiotic factors that hinder tomato production and productivity, the root-knot disease, caused by nematodes of the genus Meloidogyne Goeldi 1887, stands out as one of the main phytosanitary problems in this crop, limiting yield throughout the world (Rosal et al., 2014). Thus, this activity takes onhigh economic risk because of the high investments necessary for planting this crop (Carvalho et al., 2014).
Economically, the most significant nematode species in the country for tomato crops are Meloidogyne javanica, M. incognita, M. ethiopica and M. arenaria because of their pathogenicity, high losses, reduction of final product quality (Hunt and Handoo, 2009), broad geographical distribution, wide range of hosts (Pinheiro et al., 2014) and management complexity (Damalas and Eleftherohorinos, 2011).
Chemical control is the phytosanitary method most used by producers. Although efficient, chemical molecules are highly toxic to humans, animals and ecosystems (Damalas and Eleftherohorinos, 2011). In this context, the study of alternative measures for the control of phytonematodes has become crucial, provided that the aim is the adoption of practices that minimize pressure on the environment (Timper, 2014; Pastori et al., 2017; Bhatt and Sharma, 2018).
The benefits of silicon accumulation and its success in the management of various pathogens have already been demonstrated for several crops, e.g. Wang et al. (2017). For nematodes, success has been shown for M. javanica in bananas (Oliveira et al., 2012), rice, beans and soybeans (Mattei et al., 2015), for M. incognita in tomatoes (Melo et al., 2012), and for En-neothrips flavens Moulton 1941 in peanuts (Dalastra et al., 2011), among others.
It is known that the characteristics of silicon allow its use in management programs because of stiffening of plant cell walls and influence on the synthesis of toxins in the biochemical responses of plants and in the gene expression of defense proteins (Dannon and Wydra, 2004; Wang et al., 2017). However, despite advances in research that promote use of this new and promising form of phytonematode management in the field, little is known about which silicon dosages are more efficient in the management of this pathogen and do not negatively affect the nutritional conditions of plants.
The objective of this study was to evaluate the effect of silicon dioxide (SiO2) on the initial development of tomato plants, as well as to determine the best doses of SiO2 in the induction of plant resistance to parasitism by M. incognita.
MATERIAL AND METHODS
This study was conducted in a greenhouse located at the Center of Agrarian Sciences and Engineering of the Federal University of Espírito Santo - CCAE/ UFES, Alegre-ES (20°42' S and 41°27' W at 269 m a.s.l.). The climate of the region, according to the Kõppen classification, is type "Aw", characterized by hot and rainy summers and dry winters. The average annual temperature is 23°C, with annual rainfall around 1,200 mm.
This experiment was set up in a completely randomized design with ten treatments and five replicates in a 5x2 factorial scheme consisting of five concentrations of SiO2 (0, 0.15, 0.3 , 0.45 and 0.6 g dim3 of soil) with the presence or absence of M. incognita (zero and 1,500 second stage juveniles, J2 + eggs). The SiO2 source was the commercial product AgriSil® (98% SiO2, pH 7.5-8), and the doses were 0, 50, 100, 150 and 200%, adapted from the recommendation of Alvarez and Ribeiro (1999).
Tomato seeds, Santa Cruz variety, were planted on a tray with 128 cells containing Bioplant® commercial substrate, and the seedlings were transplanted 21 d after sowing into pots containing 4 dm3 of medium textured red-yellow latosol-based substrate. The fertility management was done according to the recommendation of Prezotti et al. (2007). Five days after seedling transplant, SiO2 was diluted in water and applied individually via irrigation directly to the pots, with the respective doses of each treatment.
Seven days after applying the product, 1,500 second stage M. incognita juveniles (J2 + eggs) were inoculated in each pot. The plants were monitored constantly to avoid the presence of diseases and pests. The control of invasive plants was done manually. The soil moisture was maintained close to field capacity, at around 60%, with daily irrigation shifts.
After 30 d of nematode inoculation, the following parameters were evaluated: plant height (PH), number of leaves (NL), shoot fresh weight (SFW), shoot dry weight (SDW), percentage of dry matter (DM %), root fresh weight (RFW), number of galls (NG), final nematode population (FP), and population per gram of root (Pgr). The nematode extraction was done according to the method proposed by Hussey and Barker (1973), as modified by Bonetti and Ferraz (1981).
The data obtained for each of the evaluated variables were submitted to analysis of variance and the doses were adjusted by regression. The means of the variables related to the presence or absence of M. incognita were compared by t-test at 5%. The normality of the data was verified using the Kolmogorov-Smirnov test (P<0.05), and Pearson linear correlation (P<0.05) was used among the variables.
RESULTS AND DISCUSSION
The variables SFW, PH and NL were significantly affected by the presence of nematodes (Tab. 1), where in the majority of the treatments, the means differed statistically, affirming that the presence of the pathogen culminates in lower development of tomato plants, with a lower weight gain. This information is consistent with results obtained by Melo et al. (2012) and Moreira and Ferreira (2015), who characterize dwarfism as one of the main symptoms of tomato nematode infections (Dias et al., 2016). In addition to having compromised growth, the infested plants had an apparent reduction of total leaf area, because of a smaller number of leaves, which negatively affected their photosynthetic capacity. Peluzio et al. (1999) and Guimarães et al. (2009) proved that fruit filling is directly related to leaf area and number of leaves since leaves behave as a source of photoassimilates to fruits, which are sinks. According to these authors, the highest gain in leaf area occurs at the initial stage of crop development and is determinant throughout the tomato crop cycle.
Table 1 ANOVA of the agronomic variables comparing their means in the presence and absence of nematodes.
SFW (kg) | (kg) | |||||||
Dose | C/N | SE | S/N | SE | C/N | SE | S/N | SE |
0% | 0.034 a | 0.0021 | 0.067 b | 0.0048 | 0.011 a | 0.0022 | 0.020 a | 0.0045 |
50% | 0.059 a | 0.0083 | 0.062 a | 0.0050 | 0.017 a | 0.0046 | 0.019 a | 0.0024 |
100% | 0.053 a | 0.0048 | 0.079 b | 0.0098 | 0.019 a | 0.0032 | 0.025 a | 0.0069 |
150% | 0.035 a | 0.0022 | 0.076 b | 0.0048 | 0.010 a | 0.0026 | 0.015 a | 0.0035 |
200% | 0.039 a | 0.0071 | 0.063 b | 0.0053 | 0.074 a | 0.0025 | 0.014 a | 0.0036 |
RFW (kg) | NL | |||||||
Dose | C/N | SE | S/N | SE | C/N | SE | S/N | SE |
0% | 0.013 a | 0.0006 | 0.012 a | 0.0029 | 7.6 a | 0.40 | 8.4 a | 0.40 |
50% | 0.014 a | 0.0017 | 0.023 b | 0.0026 | 7.8 a | 0.37 | 10.8 b | 0.97 |
100% | 0.020 a | 0.0029 | 0.018 a | 0.0018 | 8.2 a | 0.58 | 9.6 b | 0.24 |
150% | 0.013 a | 0.0014 | 0.013 b | 0.0020 | 7.4 a | 0.24 | 9.2 b | 0.37 |
200% | 0.010 a | 0.0021 | 0.019 b | 0.0028 | 7 a | 0.55 | 9.2 b | 0.37 |
DM (%) | ||||||||
Dose | C/N | SE | S/N | SE | C/N | SE | SN | S/E |
0% | 0.64 a | 0.011 | 0.65 a | 0.014 | 32.39 a | 6.81 | 28.95 a | 6.56 |
50% | 0.614 a | 0.025 | 0.73 b | 0.024 | 30.69 a | 9.55 | 30.29 a | 2.79 |
100% | 0.63 a | 0.021 | 0.80 b | 0.023 | 33.91 a | 2.87 | 30.23 a | 7.72 |
150% | 0.57 a | 0.015 | 0.78 b | 0.028 | 29.89 a | 7.14 | 19.09 a | 3.90 |
200% | 0.55 a | 0.027 | 0.72 b | 0.032 | 17.94 a | 4.30 | 21.35 a | 3.88 |
SFW: shoot fresh weight in kg; SDW: shoot dry weight in kg; PH: plant height in meters; RFW: root fresh weight; NL: number of leaves; DM (%): percentage of dry matter; C/N: plants infected by nematodes; S/N: plants not infected by nematodes; SE: standard error of the mean. Means followed by different letters differ statistically according to the t test (P<0.05).
For the variables SDW and RFW, the means of the plants infested by the pathogen were smaller than those not infested, but did not differ statistically, in the majority of the treatments. Dias et al. (2016) found similar results; thus, these variables do not explain with confidence the effects of this pathogen on plants at the initial stage of development. The mean of the DM (%) variable also did not differ statistically.
The analysis of variance of the effect of SiO2 on the tomato plants showed that plant height was significantly influenced, where the use of SiO2 resulted in higher growth. However, high doses of SiO2 cause an inverse effect, i.e., the plants presented reduced growth, which can be explained by the indirect effect of silicon on the absorption of zinc. High doses of SiO2 make the amount of phosphorus available in the soil high, precipitating available zinc in the form of zinc phosphate (Paim et al., 2006) and compromising the development of tomato plants since this nutrient is essential to the development of this crop.
Figure 1 shows the curve formed by the quadratic model that explains the growth of the tomato plants as a function of the addition of SiO2 to the soil, where the best growth is found at the maximum point of the regression curve. Thus, the dose that provided the highest height was 116.63%, equivalent to 0.34 g dm-3 of SiO2, reaching a maximum height of 0.8 m per tomato plant. Pires et al. (2009) found that tomato plants with greater height at the initial stage of development tend to be more productive.
The variables SFW and NL were not significantly influenced by the addition of SiO2; however, both variables presented a positive linear correlation with plant height (Fig. 2). Porto et al. (2014) used these variables to infer optimal nutrition conditions for tomato plants and determined that plants that receive adequate nutrition have a certain balance between the values of the mentioned variables, affirming that plants with a greater height, weight and number of leaves are better-nourished plants. Pulz et al. (2008), studying the effect of silicates on potatoes, also observed that the height of plants was greater in the presence of silicon. The same authors demonstrated that higher growth of potato plants may be associated with higher availability of phosphorus in soils with a higher silicon content. The silicates exert competition for the adsorption sites of phosphorus, causing the sites to be saturated or blocked by silicate anions, increasing phosphorus adsorption (Pulz et al., 2008). Similarly, Prado and Fernandes (2001) also observed a higher available phosphorus content in soil treated with silicate.
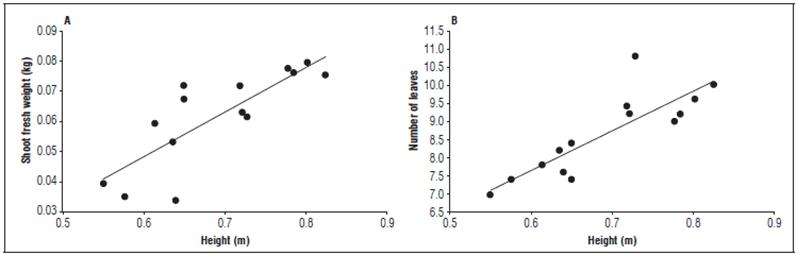
Figura 2 Correlation between plant height and the variables shoot fresh weight and number of leaves per plant. A. Pearson correlation coefficient at 5% probability (r = 0.81, P<0.0001); B. Pearson correlation coefficient at 5% probability (r = 0.83; P<0.0001).
For MFPA and MSPA, no significant correlation was observed between DM% and FP and NG. However, lower DM (%) indexes were obtained at the extremes (Fig. 3), that is, with the highest and lowest pathogen infection, which coincided with the lowest and highest doses of SiO2, respectively. Therefore, both high pathogen infection and high SiO2 doses negatively affect the development and accumulation of dry matter in these plants. Rahi et al. (1988) found that the dry and fresh biomass of tobacco plants infected with M. incognita and M. javanica were almost half of the biomass of uninfected plants.
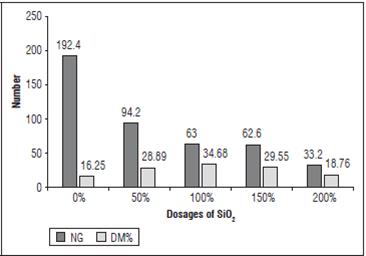
Figure 3 Relationship between the number of galls (NG) in the roots of the plants and percentage of dry matter (DM %) in the shoot at the different doses of SiO2.
The different doses of SiO2 affected the formation of galls in the roots of the inoculated plants (Fig. 4): the higher the SiO2 dose, the lower the number of galls. These results are similar to those found by Moreira et al. (2013), where the number of galls formed by M. incognita was lower as a result of the application of different doses of eugenol in a tomato crop. This author stated that the number of galls is directly associated with the amount of nematodes that can penetrate and settle inside roots. Thus, a lower number of galls also means less penetration of the pathogen in the tissues of the roots, inferring that SiO2 was able to induce resistance to the attack of the pathogen, possibly by a physical barrier formed by Si, inhibiting the penetration of the pathogen.
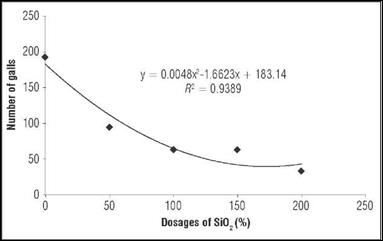
Figure 4 Effect of the different doses of SiO2 on the number of galls (NG) in the roots of tomato plants.
The final population in the roots did not show dependence on the tested SiO2 doses, so it was not possible to propose a regression model to determine silicon concentrations capable of reducing FP. However, the number of galls had a positive correlation with the final population (Fig. 5), meaning FP depends on the number of galls, increasing as the number of galls increases. This relationship occurs because galls are modified cells where gall-forming nematodes settle and extract nutrients to complete their life cycle and multiply. Therefore, the final population of the pathogen tends to be proportional to the number of modified cells present in the roots of the infested plants (Danchin et al., 2016; Ralmi et al., 2016).
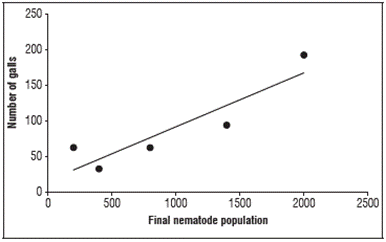
Figure 5 Correlation between the final nematode population (FP) and the number of galls (NG) in the roots of tomato plants. Pearson correlation coefficient at 5% probability (r = 0.90, P<0.035).
Another variable that was influenced by the different doses of SiO2 was Pgr. It can be observed from Figure 6 that Pgr had lower values than the control, where silicon was not added, once again showing that silicon was able to reduce penetration and the consequent multiplication of nematodes inside the root tissues.
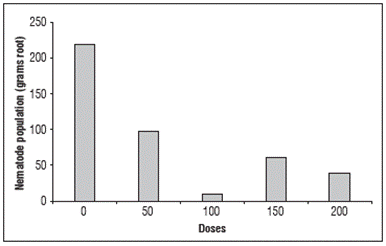
Figure 6 Relationship between the nematode population per gram of infected root and the different doses of silicon dioxide.
Several other authors have found positive results in the use of Si for the management of stresses of a biotic or abiotic origin in both tomato and other crops. Conceição et al. (2014) observed that the application of potassium silicate, alone or in combination with microorganisms antagonistic to the bacterial stain of melon fruits, reduced the severity of the disease. The disease index was reduced by 87%, and the the area was reduced by 65% when using Si with the antagonistic Rhodotorula aurantiaca Saito in the management. Cao et al. (2017) observed that Si can act as a mediator in tomato plants subjected to water stress, reducing the harmful effects. Sousa et al. (2013), studying the infection processes of Puricularia oryzae (Cooke) Sacc in wheat fertilized with Si, observed that the presence of Si reduced the symptoms of the disease in wheat leaves, emphasizing the possibly that, in the areas where the Si concentration was higher, the fungus penetrated less and had greater difficulty in colonizing. Mattei et al. (2015) observed that the hatching of M. javanica eggs was reduced in the presence of Si. In the tomato, a possible deposit of silicon in the root epidermis may have inhibited both the penetration of nematodes and their reproduction inside the cells.
The resistance to pathogen action conferred by the use of silicon is mainly related to the physical barrier formed by salicylic acid in the cells of the epidermis at the penetration points of the pathogen, improving the mechanical resistance of the cells. Silicon accumulates mainly in the cell wall, forming a cellulose-silicon bond that changes the natural barrier of the plant. It changes the anatomy and increases thickness as a result of a greater degree of silicification and accumulation of lignin and phenolic compounds in the lesions (Mendes et al., 2011), affecting the signs of recognition between the plant and the pathogen (Chérif et al., 1994), the latter being a more efficient and enduring form of the resistance mechanism. Cao et al. (2017) affirmed that the greater accumulation of Si in the roots can maintain the integrity of the cell by improving its mechanical properties after observing a higher Si content in the roots of tomato plants that received a Si application. Besides the formation of the mechanical barrier, the presence of silicon in a plant can improve the activity of plant defense enzymes, inducing the production of antimicrobial compounds and acting in the regulation of plant protection pathways, such as the routes of jasmonic acid, salicylic acid and ethylene. Moreover, it can influence the gene expression of plant defense proteins (Bockhaven et al., 2013; Bhatt and Sharma, 2018). Although the protection mechanisms provided by Si were not evaluated in this study, there is sufficient evidence in the results and in the literature that confirms Si is able to reduce the penetration and reproduction of M. incognita in tomato plants.