INTRODUCTION
Fruit trees have important adaptation strategies and tend to be more resistant to climate change (CC) because of their perennial nature (Sthapit et al., 2012). However, the CC scenario is no longer favorable for many of these species, such as for vines in Mediterranean regions where conditions stopped being favorable because of elevated air and soil (STe) temperatures (Torres et al., 2018). Lippmann et al. (2019) described global warming as one of the most damaging aspects of CC, which affects the growth and development of plants throughout the entire phenological cycle. On the other hand, Sauer and Peng (2018) highlighted the effects of global CC on temperature and precipitation patterns that have significant impacts on surface soil conditions, which in turn have significant implications for crop growth and food safety and security. According to these authors, solar radiation influences the thermal regime on Earth's surface. Bradford et al. (2019) reported that increases in STe in the 21st century were going to be relatively uniform at a geographic level and robust according to climate models.
Because of greenhouse gas emissions (GGE), global warming will exceed 2°C above pre-industrialized temperatures, and this increase will have a huge impact on the Earth and sea levels, affecting the economic and social development of all countries (Zheng et al., 2019). Increased global temperatures will have a direct effect on the structure and function of many ecosystems (Mishra et al., 2019), including impacts on the physiology and growth of trees (Ceulemans et al., 1999), affecting 35% migratory birds, 52% amphibians and 71% corals in the world (UNEP, 2009).
Because of global warming, changed rain patterns and increased frequency and intensity of extreme weather events affect food security (IPCC, 2019). The consequences for agriculture will be more severe in poor countries in the tropics and subtropics, with higher initial temperatures, on marginal or degraded lands, and in underdeveloped areas with a poor adaptation capacity (Yohannes, 2016).
Carbon dioxide (CO2), methane (CH4), and nitrous oxide (N2O) are the main gases from respiration (Surówka et al., 2020) and anthropogenic activities, which have increased the temperature of Earth's surface by approximately 1°C since 1990. The IPCC (2013) predicts that, by the end of the 21st century, the global average temperature of Earth's surface will increase by between 2.6 and 4.8°C. Among GGE, CO2 has a constant, almost linear correlation between its accumulated emissions and projected global temperatures (Surówka et al., 2020).
The IPCC (2013) predicts that alterations in precipitation patterns, together with the increase in STe, may result in warmer and drier climatic conditions and that, by the end of the 21st century, droughts at the regional and global levels, could increase in intensity and/or duration. Bhatt et al. (2019) pointed out that an increase in STe as the result of global warming will cause water stress, which will affect different parameters of yield and quality in different crops. Similarly, Kurylyk et al. (2014) reported that alterations in precipitation regimes and increases in air temperature (ATe) as the result of CC will influence the thermal regimes in subsoils, increasing edaphic and groundwater temperatures and affecting quality to the detriment of ecosystems originating from groundwater.
Bonadeo et al. (2017) stated that STe depends almost entirely on the sun and the energy mostly lost to the environment through convection, making it clear that global warming directly affects soil temperature. Patil and Kulkarni (2016) reported that the STe expresses the intensity of the heat energy available in response to the absorption of solar radiation directly or indirectly, and that STe depends on the relationship (ratio) between absorbed and lost energy.
Soils have a relatively high heat capacity, meaning they can involve considerable energy with their changes in temperature (Nobel, 2020). In the soil, the temperature varies greatly with depth and over time, while, in the different layers of the surface soil, STe fluctuates mainly in response to changes in ATe, irradiation and radiant heat transfer. Also, heat transfer by conductivity is responsible for temperature variations, with soil moisture playing an important role. The most stable temperature is in the deeper layers (Marschner, 2012). The STe at moderate depths of 1 m can be extremely stable on a daily basis, with variations below 0.1ºC, and very stable seasonally (Nobel, 2020).
In the countries of the South American Andes, many fruit crops are grown on the slopes of mountains, where ATe and STe change with altitude, e.g. ATe decreases about 0.6°C with an increase of 100 m (Fischer et al., 2021), which has a large impact on the physiological processes of plants. Fruit trees at higher altitudes develop their roots more superficially (Fischer et al., 2007) to take advantage of the higher temperature of the surface layer during midday (Fischer and Melgarejo, 2020). In addition, colder soils at altitude restrict roots as an important dumping ground for plants, as mentioned by Marschner (2012), as an important limitation for the growth of roots at high altitudes.
In a study with data from 41 meteorological stations in Colombia at altitudes greater than 500 m a.s.l., the STe at 50 cm was greater than the ATe, with the STe varying negatively and linearly with altitude, but was highly, positively correlated with the ATs (Callejas and Castellanos, 1991). Castro et al. (2016) calculated the Colombian altitudinal zones (pisos térmicos): "Warm" (less than 1,000 m a.s.l.), "Temperate" (1,000-2,000 m a.s.l.), and "Cold" (2,000-3,000 m a.s.l.), with an ATe (and STe) >24°C (>22°C), 18-24°C (15-22°C) and 12-18 (8-15°C), respectively.
Jungqvist et al. (2014) emphasized that there are complex, non-linear relationships between the responses of ATe and STe to climate change; however, despite its influence on hydrological and biogeochemical processes, Ste has received little attentionin climate impact studies even though it is equally affected by global warming. Likewise, Kath and Pembleton (2019) highlighted the impact of CC on numerous environmental and agricultural processes in the soil, which surpassed the importance of STe in biochemical and physical processes. Likewise, these authors emphasized the lack of studies on the influence of CC on STe. Sperling et al. (2017) reported that trees do not perceive a single temperature regime but are found in two thermally different environments: ATe with great changes that affect the canopy, and STe that is dampened with slower changes in the rhizosphere. Generally, Ste are somewhat lower than ATe, with some exceptions and seasonal fluctuations (McMichael and Burke, 2002).
Therefore, the objective of this review was to report the effects of soil temperature, with an emphasis on fruit trees because impacts on the establishment, growth, development and physiology of plants impact decisions related to alleviating the effects of global warming.
SOIL TEMPERATURE AND ITS INFLUENCES
Soil covers
Ground cover with different materials or mulching is used to modify the STe, which impacts humidity, increasing the water retention capacity of soil by reducing water loss through evaporation, reducing other effects such as weed infestation and certain insects-pests, increasing microbial activity, improving crop growth and yield in many cases (Amare and Desta, 2021; Cleves et al., 2012), reducing the loss of nutrients through leaching processes and erosion, and reducing damage to fruits through contact with the ground (Cleves et al., 2012). Importantly, higher humidity and temperatures increase the mineralization of organic matter in soil.
A range of different organic materials are also being used (mostly plant residues, e.g. cereal straw) that immobilize much of the air and transmit heat slowly from the surface because of a very low thermal conductivity (Bonadeo et al., 2017). Lal (2013) and López-Bellido (2015) mentioned that the use of waste mulch and other biosolids is a technique for turning soil into a carbon sink, reducing GGE. The availability of water exerts a strong influence on the development of vegetation cover (Zhu et al., 2019). Gambetta et al. (2015) evaluated the application of lime as a mechanical cover in mandarin varieties Satsuma and Clementina and found that the STe decreased by between 0.5 and 3°C.
Mulch is made with synthetic fibers, natural fibers, or with blends of both fibers, varying in thickness, color, texture and lifetime. However, the most widely used mulch for large-scale projects is plastic film made with polyethylene (PE), mainly LDPE (Amare and Desta, 2021; Rao et al., 2017). Black and blue plastic increases STe (Amare and Desta, 2021), while white and white on black can decrease STe, as compared to non-padded soil (Helaly et al., 2017).
In tropical and subtropical areas, high STe can reduce yields because of overheating of insufficiently covered soils (Fischer and Orduz-Rodríguez, 2012). In these hot areas, silver plastic (30-micron PE) has shown large reductions in STe, e.g. watermelon (Citrullus lanatus) had up to a temperature of 20.1°C, as compared to 30.4°C with black plastic and uncovered soil (32.5°C), achieving the highest yield using silver PE (Rao et al., 2017).
Similar results were found in tomatillo (Physalis pubescens), in which a white-on-black, double-sided PE covering improved plant growth and production, as compared to black plastic and uncovered soil. Helaly et al. (2017) suggested that the white color reflected a greater amount of radiation available to plants, thereby increasing photosynthetic activity.
Also, in many melon crops in warm climates in Colombia, special plastic mulch is used (Fischer and Orduz-Rodríguez, 2012), especially silver on black (Cleves et al., 2012), while strawberries in cold climates see the use of black plastic (PE, 1.2 gauge) (López-Valencia et al., 2018). Bakshi et al. (2014) compared black and transparent PE films with organic mulch materials (paddy straw, wheat straw and dry grass) in strawberry, where the best results for productivity were obtained with two plastic mulch materials. In any case, the effect of different types of mulch on the STe may vary according to the study area and the investigated crop (Amare and Desta, 2021).
Vegetation generally has the same effect as an inert cover, reducing daily and seasonal fluctuations in temperature (Bonadeo et al., 2017). Also, Daryanto et al. (2018) reported increases in STe according to cover crops but found an aggravating factor in an increased ability for nematodes to infect the roots of the main crop. In addition, associated crops influence STe.
The soil of coffee intercropped with banana in Brazil reduced its temperature by 5°C, increasing the moisture content of the soil by 17% as the result of the shading effect of the intercropping (Silva et al., 2020). On the other hand, managing tree crowns with pruning, as studied in cacao, in an open canopy, allowed greater penetration of solar rays to the ground, which increased the STe by 2.1°C, the ATe by 2.5°C, and the vapor pressure deficit by 0.55 kPa in the microclimate of the trees, as compared to closed canopies (Jiménez-Pérez et al., 2019).
In addition to soil cover, the formation of raised furrows or mounds, in which fruit trees are planted (or hilled), can increase STe by about 1.5 to 3°C, which is especially favorable in germination. The initial growth of plants and the addition of organic matter to these furrows reduce abrupt diurnal changes in temperature and increase the availability of nutrients (Fischer and Torres, 1999).
In general, soil management practices modify the climate and temperature near the surface because the properties of the soil are crucial for the diffusion of incoming energy that is distributed, heating the soil, increasing evapotranspiration and warming of the air above the soil surface (Sauer and Peng, 2018). Patil and Kulkarni (2016) listed other factors that also influence STe, such as structure, texture, irrigation, drainage, compactness of water retention and topography, as well as the presence of cover crops (McMichael and Burke, 2002).
Water
Soil moisture is the amount of water in the active layer of the soil, typically the top 1-2 m. It is extremely important because it is the main source of water for agriculture and natural vegetation. Soil moisture is defined as the volume of water required for plant growth and development and is temporarily available (Houser, 2010; Deng et al., 2016). Because of the higher heat capacity and thermal conductivity of water, as compared to air, a wet soil cools faster than a dry one, likewise, the use of radiant energy in the evaporation of water decreases the STe. Consequently, wet soil, either from rain or irrigation, is colder than dry soil (Fischer and Torres, 1999). So, moist soil should conduct heat better than a dry one; however, on a sunny day, the surface of a dry soil heats up more quickly, and, during the night, with a clear sky, soil cools much faster than with a humid surface (Bonadeo et al., 2017). Likewise, these authors also clarified that the greater fluctuation of temperature on a dry soil surface is dampened with depth; so, at about 7 to 10 cm, there is little difference in the edaphic temperature because of humidity.
An important aspect of agriculture in the tropical highlands is seen with a greater capacity and thermal diffusivity of water within a certain temperature range, where humidity increases the STe in the day and protects the grounds during nights with a clear sky, which can cause rapid cooling, thereby reducing susceptibility to frost, as reported by Fischer and Torres (1999).
For water uptake by the roots, the maintenance of the fluidity and semi-permeability of the membranes is key to functioning, preventing STe from affecting the rate of water absorption, influencing aquaporins, which are proteins that regulate the movement of water through the cell membrane (Lambers and Oliveira, 2019; Vera-Estrella et al., 2004).
Microbial processes
The STe, in conjunction with water and the availability of nutrients in the soil, influences the speed and development of microbial processes. Some of these processes cause the decomposition of organic matter (Fig. 1) and denitrification and nitrification, which control the storage of carbon in the soil and affect the exchange of GGE between the soil and the atmosphere (Mu et al., 2013, Bonadeo et al., 2017). That is, an increased STe contributes to increases in the emission of these gases into the atmosphere. Thus, the activity of thermophobic and thermophilic microorganisms depends on STe, and most have an optimal activity in the range of 25 to 35°C (Patil and Kulkarni, 2016). Therefore, the organic carbon sequestered in soil is depleted when an increased STe increases the mineralization rate, where López-Bellido (2015) pointed out that, with each 10ºC increase in STe, mineralization doubles, increasing the detrimental effect of CO2 in the atmosphere as GGE.
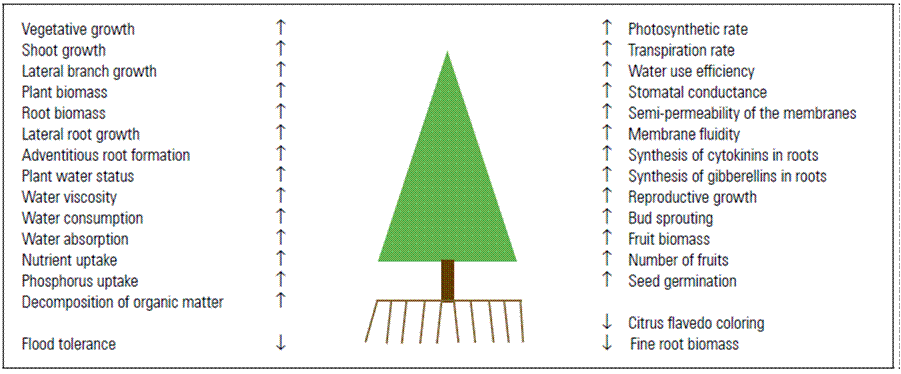
Figure 1. Diagram on the increase (↑) and reduction (↓) of growth parameters and the physiology of the fruit plants under the effect of an increased soil temperature, within the minimum (about 8-15ºC) and maximum (about 32-43ºC) ranges, depending on the species.
Fischer and Orduz-Rodríguez (2012) pointed out that, starting at 35°C, the symbiosis of roots with rhizobia and mycorrhiza ends. The responses of fungi to environmental factors depend mainly on the strain; in most cases, an increase in STe favors mycorrhizal colonization in roots and the spread of hyphal networks (McMichael and Burke, 2002), while drought reduces colonization by arbuscular mycorrhizal fungi (AMF) (Torres et al., 2018).
Increases in the rate of root respiration increase the emission of CO2 into the atmosphere at a higher STe, expending more of the carbons produced in photosynthesis (Lambers and Oliveira, 2019), just as edaphic microbial respiration releases more CO2 into the atmosphere under these conditions (Marschner, 2012). Thus, the increase in STe because of global warming increases GGE; however, a higher STe, up to a certain degree, means trees grow and photosynthesize more, accumulating a greater amount of carbon (Pumpanen et al., 2012).
Plant growth and development
Soil is a very important component in the energy balances of plants. Thus, short-wave irradiation can be reflected from the soil surface, which is the source of long-wave radiation that can correspond to a temperature very different from that of air, and heat can be conducted to or from stems near the ground (Nobel, 2020). In addition, these authors stated that soil can store considerable amounts of energy, unlike leaves. This indicates that STe can significantly affect plant growth and development. Also, McMichael and Burke (2002) emphasized that STe changes have an important impact on the growth and development of plant roots and, consequently,on total plant productivity.
Climatic and edaphic factors markedly affect the aptitude of plants, that is, their establishment, development, reproduction and survival (Taiz et al., 2017), where temperature is the main factor that controls the growth and development rates of plants (Sawan, 2018). In general, initial growth processes, such as seed germination (Fig. 1) and seedling emergence, are highly sensitive to STe, while plant growth and development, extension and growth of the radical system and fruit maturation depend a lot on ATe but also on changes in STe (Zhang et al., 2019). Thus, Patil and Kulkarni (2016) ranked STe as the first control of plant growth, more important than ATe in the initial phase.
Likewise, STe affects important processes in crops, such as the absorption of water and nutrients and the synthesis of hormones in the roots (Fig. 1) (Fischer and Ordúz-Rodríguez, 2012). It is well known that roots are a site for the synthesis of hormones, mainly cytokinins (Lambers and Oliveira, 2019) and gibberellins, which control growth by cell division and elongation, respectively (Taiz et al., 2017).
Sawan (2018) pointed out that the balance between the vegetative and reproductive development of plants is not only influenced by fertility, soil moisture and distance between plants but also by STe. Thus, Callejas et al. (2009) reported that among the factors that affect the development of the roots under optimal conditions, STe is the main modifying agent for radical growth.
For many tropical species, the optimum soil temperature range is between 20 and 25°C but should not exceed 30 to 32°C (Marschner, 2002). For fruit trees, Agustí (2010) recommended a STe range between 10 and 30ºC, while lower and higher temperatures markedly reduce the development of the root system.
Lambers et al. (2008) confirmed that the exposure of roots to low temperatures reduces the extension of the root system, depending on the species. This confirms the report by Marschner (2012), who asserted that the optimum temperature for root growth is under low genetic control. At very low edaphic temperatures (<10°C), the absorption of nutrients is very limited (Patil and Kulkarni, 2016), especially for phosphorus, which is transported to the roots by diffusion. Likewise, roots that grow with a low STe have a reduced permeability of the membranes, and viscosity of the liquids increases, reducing their transport in the root system and their entry into the stem xylem (Fischer and Orduz-Rodríguez, 2012).
Because nutrients have different transport proteins and transport pathways (symplastic and apoplastic), an increase in the temperature of the root zone (TRZ) can result in an increase in the concentration of potassium, NH4 +, NO3-, and PO4 3- , apparently because of an increase in root respiration as a function of temperature (Ahmad and Prasad, 2012), but a reduction in calcium in the xylem exudate. On the contrary, lowering the STe can affect the availability of iron and zinc (Marschner, 2012). In turn, Lambers et al. (2008) pointed out that a reduced rate of nutrient absorption at a low TRZ is, at least in part, a response to the lower demand for nutrients by the plant, under these conditions.
The optimum temperature for root growth tends to be lower than that of the stem, which is why, after a cold season, roots begin to grow earlier than leaves (Lambers et al., 2008). While temperate climate species have an optimum temperature for their roots between 10 and 30°C, those of the subtropics inhibit radical growth <10-15°C (Lambers et al., 2008). Marschner (2012) mentioned that a low TRZ inhibits stem growth more than roots, leading to a high root/stem rate.
Root respiration spends 10 to 50% of the total carbon assimilated each day by photosynthesis, thereby increasing the TRZ increases the allocation of carbohydrates for root respiration, which is the driving force for the growth and maintenance of the roots and for the absorption and transport of ions to the xylem (Lambers et al., 2008).
Biomass accumulation in Pinus silvestris was higher when increasing the STe. In addition, net photosynthesis and stem and root respiration (Fig. 1) increased with the edaphic temperature (Pumpanen et al., 2012). Ahmad and Prasad (2012) reported that a high STe acclimatization response is to maintain a lower respiration in the roots and therefore reduce the expenditure of carbohydrates during respiration. On the other hand, Marschner (2012) stated that root respiration decreases in cold soils because low temperatures slow down the enzymatic activity of this process.
EXAMPLES OF STUDIES IN FRUIT TREES
In fruit trees, root studies have shown a growth pattern that depends on the crop, age, climate and environmental stress (Callejas et al., 2009), confirming the findings of Marschner (2012) that STe has a substantial effect on root and plant growth. Likewise, studies have shown that the conditions that control shoot growth in fruit trees are related, at least in part, to STe (Sthapit et al., 2012).
For propagation in fruit trees, STe has important effects. For example, green cuttings, such as in the Malus, Prunus and Pyrus species, form adventitious roots with a TRZ that can exceed 25°C, and rooting can be benefited by cooling leaves at the same time with intermittent water misting (Friedrich and Fischer, 2000). For the germination of melon seeds, Fischer et al. (1997) reported minimum, optimal and maximum STe as 13-19, 30-40 and 45-50°C, respectively, while Agustí (2003) found optimal temperatures of between 31 and 34°C for the germination of citrus seeds. The germination process is very sensitive to STe because the imbibition of water requires enzymatic and respiratory activity that depends on each species with a certain Q10 (Bonadeo et al., 2017).
Studies on the influence of STe on plant growth should consider depths at a greater proportion of the roots. In the case of herbaceous fruit trees such as strawberry and melon, the depth is between 5 and 25 cm (Fischer, 2012), as compared to woody trees that root up to 80-100 cm (Agustí, 2010).
In grapes, Mahmud et al. (2018) observed that stem shoots were a more preferential sink than roots at a TRZ of 30°C, which reduced root growth by half (Tab. 1) This confirms that the optimal STe for stem growth is higher than for roots (Lambers et al., 2008). In cape gooseberries, a TRZ of 29°C significantly promoted the growth of lateral branches (Fig. 1) on the 4 main stems; however, these stems decreased their biomass at 30°C, as compared to 22°C TRZ (Fischer et al., 1997). Probably, the increase in the TRZ promoted the synthesis of cytokinins in the roots (Lambers and Oliveira, 2019), which activate the lateral sprouting of branches (Fischer and Torres, 1998), where longitudinal growth could be promoted by a higher production of gibberellins in the roots (Fig. 1) at an optimal TRZ (Agustí, 2003).
Table 1. Some studies on the effect of soil temperature on fruit trees.
Species | Effect | Authors |
---|---|---|
Grape (Vitis vinifera, cv. Shiraz and V. champinii cv. Ramsey) | In 31 cm high pots, the root growth rates of the two species decreased by 50% at 30°C TRZ relative to 22°C, while the stem shoot growth rates were greater than 30°C TRZ. | Mahmud et al. (2018) |
Satsuma 'Iwasaki' and Clementina 'Clemenpons' (on citrange 'Carrizo') and Satsuma 'Okitsu' and 'Clementina de Nules' (on P. trifoliata) | Lime was applied 2-4 months prior to harvest on the soil. The treatments decreased the STe between 0.5 and 3°C. It was possible to advance the coloration of the fruit and the harvest because they matured 2 months later than Satsuma when the STe remained below the thresholds only in Clementinas. | Gambetta et al. (2015) |
Cape gooseberry (Physalis peruviana) | With TRZ of 14, 22 and 30°C the highest accumulation of DM of the plant was obtained at 22°C, with higher DM of fine roots at 14°C, but DM of fruits was greater than 30°C. Pn and WUE increased up to 30°C, while the transpiration rate was the highest at 22°C. | Fischer et al. (2000) |
Soursop (Annona muricata) and pond apple (A. glabra) | Trees in containers at a TRZ of 5, 10, 20, 25 or 35°C were flooded or not, which produced the maximum growth of soursop at 35°C when not flooded and at 25°C with flood conditions. The combination of floods and TRZ of 5 and 10°C resulted in the mortality of trees of both species at 4 weeks. | Ojeda et al. (2004) |
Avocado (Persea americana) cvs. Velvick and Duke 7 | At 13°C TRZ, the lowest DM of roots and stems was produced, as compared to 18, 23 and 28°C. Stomatal conductance (gs) at 13°C TRZ of ‘Duke 7’ decreased more rapidly during the morning than in ‘Velvick’. | Whiley et al. (1990) |
Olive (Olea europaea) | During the months of the cold season in Spain, olive trees with STe >10°C had no effect on the water status of the plant, while, between 10 and 6.5°C, there was a linear relationship between the water status of the plant and STe. While at <6.5°C TS, the wind and relative humidity determined the water status of the plant. | Centeno et al. (2018) |
Prickly pears (Opuntia ficus-indica) | The TRZ range for root elongation was 12-43°C, depending on the day/night ATe: at 15/5°C, the optimal TRZ was 27.1°C; at 25/15°C, it was 28.4°C, and, at 32/25°C, it was 30.9°C. | Drennan y Nobel (1998) |
To alleviate the negative effects of global warming and the consequent restriction of water on the vine, Torres et al. (2018) recommended inoculation with arbuscular mycorrhizal fungi in some clones of 'Tempranillo' vines. In the vineyards of the Copiapó and Huasco valleys in Chile, the mean annual soil temperature was not related to the maximum intensity of annual growth of the 'Thompson Seedless' roots, but, rather, the annual root growth was positively correlated with the thermal diffusivity of the soil. Callejas et al. (2009) recommended soils with high thermal diffusivity as optimal for the development of root systems.
Strangely, in the cape gooseberry, a typical, tropical cold climate plant (Fischer and Melgarejo, 2020), the highest accumulation of dry mass in fruits, net photosynthetic rate (Pn) and water use efficiency (WUE) (Fig. 1) occurred at a TRZ of 30°C, meaning a low Pn and WUE at 14°C TRZ could have been related to a reduced absorption of water and phosphorus at this temperature (Fischer and Torres, 1998; Fischer and Lüdders, 1999), crucial elements in plant photosynthesis (Taiz et al., 2017). Furthermore, the acclimatization process of the plant at this lower temperature could affect the photosynthetic rate (Kochhar and Gujral, 2020).
At 14°C TRZ, fine roots (Fig. 1) produced the highest biomass (Fischer et al., 2000) (Tab. 1), possibly to explore more favorable soil spaces. As Lambers et al. (2008) pointed out, plants can invest relatively more biomass in roots when temperatures are suboptimal. Thus, the water absorption by the roots was lower at 15°C STe than at 22 and 29°C (Fischer and Lüdders, 1992). A favorable reduction in TRZ was seen at night, a situation that is more adapted to the natural environment in the field, as found by Fischer and Lüdders (1992) at 22/14°C TRZ day/night, which favored the biomass production of plants, as compared to a constant TRZ of 14, 18 and 22°C, possibly because of a lower demand for carbohydrates from the root system as the result of a reduced TRZ at night (Marschner, 2012).
Furthermore, Fischer and Lüdders (1992) found a very poor vegetative and reproductive growth at 8°C TRZ in cape gooseberries; however, at this temperature, the water consumption to form 1 g of dry mass (DM) of fruit was the lowest (1.8 L g-1) (Fischer and Lüdders, 1999), possibly an adaptation to cold temperatures and low precipitation in high altitudinal areas (up to 2,800 m a.s.l.) in Colombia (Fischer and Melgarejo, 2020). In any case, the cooling of roots at such low temperatures markedly affects the elongation of the stems, branches and leaves, which Marschner (2012) associated with an increase in the ABA concentration in the leaves. McMichael and Burke (2002) reported a reduced uptake of water and nutrients under suboptimal STe conditions.
In Citrus, not only a low ATe but also the STe plays a key role in the coloration of the fruit peel, especially at a STe below 20°C (Lado et al., 2018). In the case of reducing the STe earlier than normal, e.g. with an application of lime as a soil cover, it was possible to advance the degreening and the harvest in Clementina grafted on citrange 'Carrizo' or P. trifoliata, while, in Satsuma, this treatment could not anticipate the color change because the STe remained above 23 and 18°C, below which the root activity of citrange 'Carrizo' and P. trifoliata is reduced, respectively (Gambetta et al., 2015).
The same effects of lowering the STe in citrus fruits was evaluated by Mesejo et al. (2012), using reflective plastic meshes placed on structures below the tree canopy. On the other hand, in citrus fruits, buds sprout at any time of the year as long as the STe exceeds 12°C (Agustí, 2003). Likewise, in these species, the degreening of the fruit rind and the synthesis of carotenoids take place at a low STe (Fig. 1), combined with a cold ATe (<15°C) (Agustí, 2003), possibly because of the inhibition of the action of cytokinins, where synthesis and export in roots are decreased (Marschner, 2012), and activation of the synthesis of chlorophylls is no longer possible.
Pond apple and soursop (Annonaceae) did not (Tab. 1) have interactions between these three factors when exposed to TRZ between 5 and 35°C and flooded. Non-flooded pond apple responded with its maximum growth with TRZ of 25 to 35°C and 20 to 25°C for flooded plants, while, for soursop, these temperatures were 35 and 25°C, respectively (Ojeda et al., 2004). This confirms that a high TRZ increases radical respiration rates (Fig. 1), but a reduced oxygen content in hot water decreases plant growth (Marschner, 2012). Under field conditions in northern Chile, the maximum growth of new roots (Fig. 1) in cherimoya (Annona cherimola) was observed by Ibacache et al. (1999) with a STe above 20°C.
In the two annonaceous species (Tab. 1), the net CO2 assimilation decreased to almost zero within the week following exposure to a TRZ of 5 or 10°C and became consistently negative during the remainder of the study (Ojeda et al., 2004), which possibly pointed to mediation by ABA to stomatal conductance when roots are under stress conditions (Taiz et al., 2017). Furthermore, the low TRZ affected the absorption of nutrients and water (Fischer and Torres, 1998), important elements in photosynthesis. The very harmful behavior of plants at a TRZ of 5 and 10°C, which caused death, especially in flooded ones, coincides with that reported by Lambers et al. (2008) for tropical species disturbances that occurred at temperatures equal to or lower than 12ºC. Likewise, flood conditions cause stomatal closure, with reduced water absorption seriously affecting photosynthesis, as observed by Villarreal-Navarreteet al. (2017) in cape gooseberries and as measured by Ojeda et al. (2004) in these two annonaceous species.
In avocados, there is little research on TRZ for growth and physiology. In a pioneering study by Yusof et al. (1969), it was found that, for Mexican avocado seedlings, the TRZ range of 21 to 27°C was the best for growth, and higher temperatures impaired it. Whiley et al. (1990) observed a harmful effect from a TRZ of 13°C (with an air temperature between 18 and 23°C) on the growth of avocado roots and stems regardless of the cultivar (Tab. 1). This low temperature was very close to 12°C, which is reported as harmful for tropical species. In addition, the reduced stomatal conductance (gs) at this TRZ could have been generated by the affectation of the fluidity of the root membranes and points to possible mediation by the ABA for the gs (Lambers et al., 2008).
In avocados, several studies have reported on the effect of STe on the incidence of diseases for the root system. For example, in a study on rootstocks inoculated with Phytophthora cinnamomi at STe of 17 and 28°C, it was found that 'Tepetl' showed a high presence of mycelium in the xylem at 17°C, which is susceptible to the pathogen, while 'Atlixco' developed a low mycelial content at 28°C, making it tolerant (Andrade-Hoyos et al., 2015). When studying the influence of the ENSO phenomenon (El Niño - La Niña) on the incidence of P. cinnamomi and Verticillium sp. in Hass avocadoes in the Department of Antioquia (Colombia), Ramírez-Gil and Morales-Osorio (2018) did not find significant results for the relationship between the amount of inoculate of these two pathogens or roots necrotized by hypoxia-anoxia with STe or ATe.
In olive trees, Centeno et al. (2018) indicated that a low STe (<6.5°C) produces high resistance to water movement, while relative humidity and air wind control the drying process in leaves. Supposedly, at STe<10°C, the hydraulic resistance of the soil to the trunk increases, generating an imbalance between the absorption of water by the roots and water lost through transpiration in leaves, resulting in a decrease of Ψs. For the reaction of olive trees to a low STe, Pérez-López et al. (2010) stated that, at STe<15°C, these trees behave similar to when they suffer from soil water deficit conditions, regardless of whether the soil water content is limiting or not.
In CAM-type fruit trees (Crassulasean Acid Metabolism), such as prickly pears, which experience large changes in ATe and STe during the day and at night in their desert and semi-desert habitats (Taiz et al., 2017). Drennan and Nobel (1998)) found an interaction between ATe and STe, that is, the ATe increased at 30/25°C day/night, and the optimal TRZ for root elongation (Fig. 1) also increased at 30.9°C (Tab. 1). Thus, the optimal TRZ for the radical growth of prickly pears was recorded between 27 and 30°C, similar to other CAM plants that root very superficially in warm regions (Drennan and Nobel, 1998). The high diversification and specification of CAM plants (including cacti and pineapple) are not only due to their adaptation to a wide TRZ range, between 12 and 43°C (Tab. 1), but also due to their adaptation to limited water environments thanks to mechanisms that open their stomata on cold nights and close them during hot and dry days (Taiz et al., 2017).
CONCLUSIONS
The effects of global climate change on temperature and precipitation patterns have significant impacts on the surface horizons of soils, which, in turn, have significant implications for the establishment, growth and production of crops and food security and sovereignty.
As compared to ATe, attention to the increasing STe in climate impact studies has been less.
An increasing STe, which is highly influenced by incident radiation and ATe, promotes root and whole plant growth and increases water and nutrient absorption, root hormone synthesis, photosynthesis, transpiration and efficient water use.
A low STe promotes plant tolerance to flooding and coloration in citrus fruits.
Soil covers, whether organic materials (plant residues) or different colored plastic films, modify the STe, as needed.
The effects of STe are specific to fruit species, where those from temperate zones tolerate lower temperatures better than species in the tropics and subtropics. Future efforts are necessary to combine genetic modifications of high yielding germplasm with appropriate management practices that could alleviate the thermal stress some fruit crops are currently suffering.