INTRODUCTION
Crops such as spearmint (Mentha spicata L.) are high added-value agricultural production alternatives; however, the global planted area is small (Karkanis et al., 2017). Spearmint is a perennial and aromatic herb in the Lamiaceae family, native to southern Europe and northern Africa (Pedraza and Henao, 2008). It is used fresh in the form of dried or powdered leaves as a condiment and herb or as herbal tea (Cirlini et al., 2016). Its oil is in demand in the pharmaceutical and cosmetic industries, ranking among the ten most commercialized extracts globally (Chrysargyris et al., 2017). In Colombia, the production of export quality spearmint is limited by low volumes that satisfy demand and comply with the norms of good agricultural practices (Pedraza and Henao, 2008). The availability of nutrients and fertilization management are two critical factors that affect crop productivity and some physiological processes, where photosynthesis stands out (Karkanis et al., 2017). The deficiency of nutrients, such as nitrogen (N), phosphorus (P), potassium (K), calcium (Ca), magnesium (Mg), sulfur (S), and iron (Fe), alters functions in the photosynthetic apparatus by decreasing the photochemistry efficiency of Photosystem II (PSII) and modifying the values of chlorophyll parameters (Smethurst et al., 2005). Nitrogen, P, and K are essential nutrients for plants that are needed to complete the life cycle (Carstensen et al., 2019). Nitrogen is the main mineral nutrient needed for chlorophyll production, which is essential for the photosynthesis process and other components of plant cells. Crop yield is affected by the N content of plants; thus, optimizing nitrogen fertilization has become an object of intense research because of its environmental and economic impact (Muñoz-Huerta et al., 2013). Phosphorus is a crucial element in ATP and NADPH generation, nucleic acid synthesis, membrane stability, enzyme activation/inactivation, glycolysis, respiration, and photosynthesis (Hawkesford et al., 2012). Potassium influences enzymatic activity and controls stomatal movement, and its deficiency causes early leaf senescence, resulting in low crop yield (Zhao et al., 2016). The requirements of N, P, and K and management of these nutrients have become a focus of research in terms of their form and absorption rate (Pandey et al., 2015). Therefore, early detection of nutrient deficits is essential for the sustainable management of natural resources available in agricultural systems, providing a good indicator of plant stress. The fluorescence emission of chlorophyll a in PSII (ChlF) has been used as a reference to determine the response of plants to the availability of different mineral nutrients (Cendrero-Mateo et al., 2015). Recent studies have shown that a ChlF analysis can be used as a sensitive indicator of deficiencies of N (Muñoz-Huerta et al., 2013), P (Carstensen et al., 2019), and K (Wang et al., 2015; Zhao et al., 2016) in different plant species.
The ChlF analysis is one of the most widely used techniques to study the photochemical efficiency and thermal dissipation of absorbed energy during the photosynthetic process (Guidi et al., 2019). The ChlF measurement is a quick and non-invasive indicator of plant photochemistry. This procedure has been used as a helpful indicator to monitor a wide range of biotic and abiotic stresses in higher plants because it provides information on the identity of various pigments, their organization, and various specific electron transfer reactions in PS II (Kalaji et al., 2016; Carstensen et al., 2019; Sánchez-Reinoso et al., 2019).
The ratio of variable fluorescence (Fv) and maximum fluorescence (Fm), expressed as Fv/Fm (Qy), has been used as an indicator of quantum yield for PSII (Rodríguez et al., 2015); therefore, it is a reference for photosynthetic activity in plants. A decrease in this ratio indicates a reduction in PSII efficiency (Guidi et al., 2019). On the other hand, the introduction of ChlF analyses to dissipation processes has meant an additional advance in detecting PSII photoinhibition. Few studies have been developed regarding the use of ChlF analysis in spearmint cultivation. The photosynthetic rate, stomatal conductance, internal concentration of CO2, and ChlF (in terms of Qy) are affected by saline stress (Chrysargyris et al., 2019a) and applications of P (Chrysargyris et al., 2019b). Therefore, this method is a potential indicator of the balance of nutrients that define plant productivity and is of great importance to responding to the technological demands of horticulturists in Colombia. The objective of this study was to identify how NPK fertilization influences the efficiency of the photosynthetic apparatus and photochemistry during the different stages of vegetative growth in spearmint.
MATERIALS AND METHODS
Location: This study was developed at the La Selva Research Center of the Corporación Colombiana de Investigación Agropecuaria (AGROSAVIA), located in Rionegro, Antioquia, Colombia, at 6º 12' 96´´N and 75º 41' 40' W, and 2,117 m a.s.l. During the evaluation period (2021/04/01 to 2021/07/31), the climatic conditions included a maximum temperature of 27.1°C, a minimum of 8.4°C, and an average of 17.3°C, with a cumulative precipitation of 396.4 mm (Fig. 1).
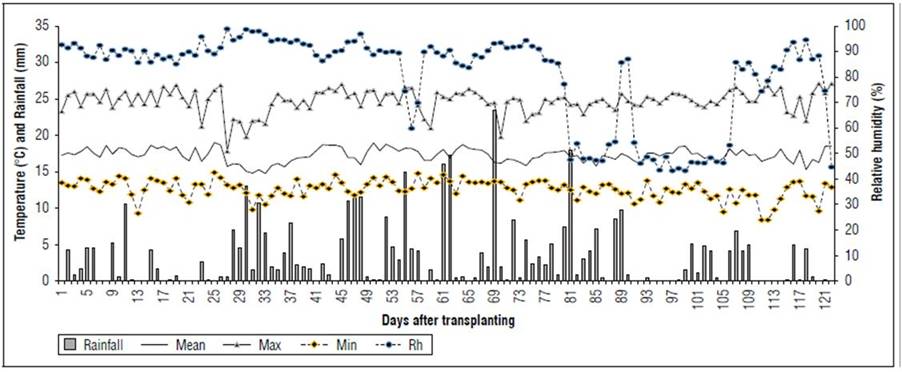
Figure 1. Daily rainfall, mean temperature (Mean), maximum (Max) and minimum (Min), and relative humidity (Rh) in a mesh house from 04/03/2021 to 07/31/2021. La Selva Research Center, Rionegro, Antioquia, Colombia.
Plant material: Spearmint cuttings were used, previously rooted with a 10 cm long stem and four pairs of leaves, which were transplanted into 5 kg bags (40 cm high × 20 cm wide). The substrate was a mixture of soil (70%), sand (20%), and coffee husk (10%), with a pH of 5.5 and concentrations of OM (18 %), P (7 mg kg-1), B (0.09 mg kg-1), K (0.69 cmol kg-1), Ca (3.4 cmol kg-1), and Mg (1.3 cmol kg-1). The substrate was disinfected with 50 g of Dazomet 98%®.
Experiment design: A randomized complete block design with five treatments and five replicates was used. The treatments consisted of five doses of chemical fertilization with the 10-30-10 (N-P-K) formula at 0, 60, 90, 120, and 180 kg ha-1. Each experiment unit consisted of 20 plants. The treatments were applied at two times: half the dose at 15 days after transplant (DAT), and the other half at 30 d after the initial application. The fertilizer application was made to drench the substrate directly in each plant, and the equivalent dose per plant was 2.2 g (180 kg ha-1), 1.5 (120 kg ha-1), 1.1 (90 kg ha-1), and 0.73 (60 kg ha-1).
Variables evaluated: Starting 14 DAT and up to 112 DAT, 10 simultaneous measurements of gas exchange and ChlF were taken weekly in the fully expanded upper leaves between 9:00 a.m. and 11:00 a.m. With the same frequency, 11 measurements of the accumulation of dry matter in the roots, stems, and leaves were taken during the vegetative stage of the plant.
Net photosynthesis (A), transpiration (E), stomatal conductance (g s ) and leaf temperature (Tleaf) was recorded in the youngest fully expanded leaves, with a photosynthetic photon flux density of 1,100 μmol m−2 s−1 using a portable LCpro ADC® (BioScientific Ltd. Hoddesdon, UK) that was conditioned with an external halogen ADC® lamp (Bioscience Ltd, Hoddesdon, UK).
The variables associated with ChlF were determined with a Fluorpen FP100® fluorometer (Photon System Instrument, Drásov, Czech Republic). Measurements were taken on leaves kept in the dark for 30 min, which were evaluated for quantum yield (Qy), Non-photochemical quenching (NPQ), and photochemical quenching (qP).
The dry matter was quantified in the leaves (LDM), stems (SDM), and roots (RDM), where each organ was placed separately in a Memmert UL 80® oven (Memmert GmbH + Co. KG, Büchenbach, Germany) at 70°C until reaching constant weight. The final weight was the biomass of the structures and their sum, recorded as the total dry weight of the plant (PDM).
The leaf area reached by each plant (LA) was estimated with a Li-3000C® leaf area meter (Li-Cor Biosciences, Lincoln, NE).
Statistical analysis. Variations between the different treatments were analyzed using a one-way ANOVA at each evaluation time. Multiple comparisons were made with a Tukey test with a probability of 5% using the “Agricolae” package in R project version 4.1.0 (R Core Team, 2017).
RESULTS AND DISCUSSION
Gas exchange. The NPK doses showed significant differences (P<0.05) for the variables A, E, and g s . In the first two evaluations (14 and 21 DAT), an irregular behavior was observed for A, E, and g s between the treatments, without following a trend as a function of the increasing NPK doses (Fig. 2A, B, C). It was evident that, after 35 DAT, the 120 and 180 kg ha-1 treatments presented the highest A, surpassing the 60 and 90 kg ha-1 treatments by up to 71% (105 DAT) and the 0 kg ha-1 treatment by 148% (91 DAT). The highest A values were recorded in the treatment with 180 kg ha-1, with the lowest in treatment 0 kg ha-1 (Fig. 2A). For E, starting at 35 DAT, the 180 kg ha-1 treatment had values that did not differ from 120 and 90 kg ha-1. On the contrary, the plants subjected to a low dose (60 kg ha-1) or no dose (0 kg ha-1) of NPK presented the lowest transpiratory rates (Fig. 2B). For g s , the treatment with 0 kg ha-1 did not differ significantly from the treatments with 180 and 120 kg ha-1 at 35, 56, and 98 DAT, presenting values similar to the treatments with 60 and 90 kg ha-1 (Fig. 2C).
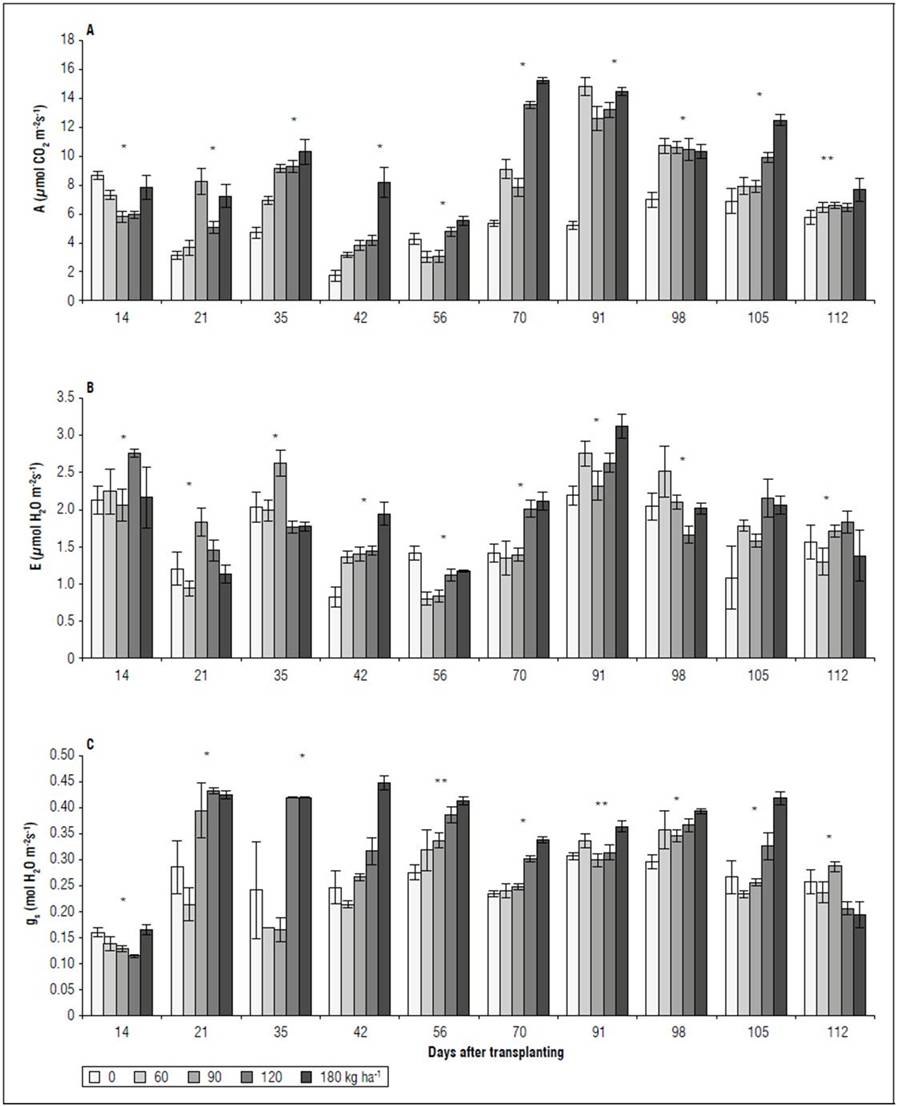
Figure 2. Effect of NPK doses on net photosynthetic rate - A (A), transpiration rate - E (B), and stomatal conductance - g s (C) of recently fully-expanded spearmint leaves. Bars represent mean ± standard error.
Chlorophyll fluorescence. There were significant differences for the variables Qy, NPQ, and qP. It should be noted that, in the first two evaluations (14 and 21 DAT), a variable behavior was observed for Qy, NPQ, and qP, with no significant difference for the increasing NPK doses. Starting at 35 DAT, the plants without chemical fertilization (0 kg ha-1) presented the lowest Qy, with values between 0.63 (DAT 42) and 0.78 (DAT 91) (Fig. 3A). The treatments with 60, 90, 120, and 180 kg ha-1 did not show differences for Qy in response to the NPK doses. The NPQ showed a trend contrary to the values of Qy. From 21 DAT, the 0 and 60 kg ha-1 treatments were characterized by the highest values for this variable, departing from the 60, 90, 120 and 180 kg ha-1 treatments, which did not present statistical differences (Fig. 3B). The qP varied over time, where, between 14 and 56 DAT, the treatments with 0 and 60 kg ha-1 presented higher values than the treatments with 120 and 180 kg ha-1. However, after 63 DAT, the 0 kg ha-1 treatment had the lowest qP, differing from 180 kg ha-1 in several evaluations, but was similar to the treatments with 60, 90, and 120 kg ha-1(Fig. 3C). For Tleaf after 56 DAT, there was a significant difference between the treatments, where the 0 kg ha-1 leaves reached the highest temperature, followed by the treatments with 60, 90, and 120 kg ha-1. The 180 kg ha-1 treatment presented the lowest value, which fluctuated between 2 and 5°C below the temperatures recorded in the 0 kg ha-1 treatment, and between 1 and 4°C below those in the 60, 90, and 120 kg ha-1 treatments (Fig. 3D).
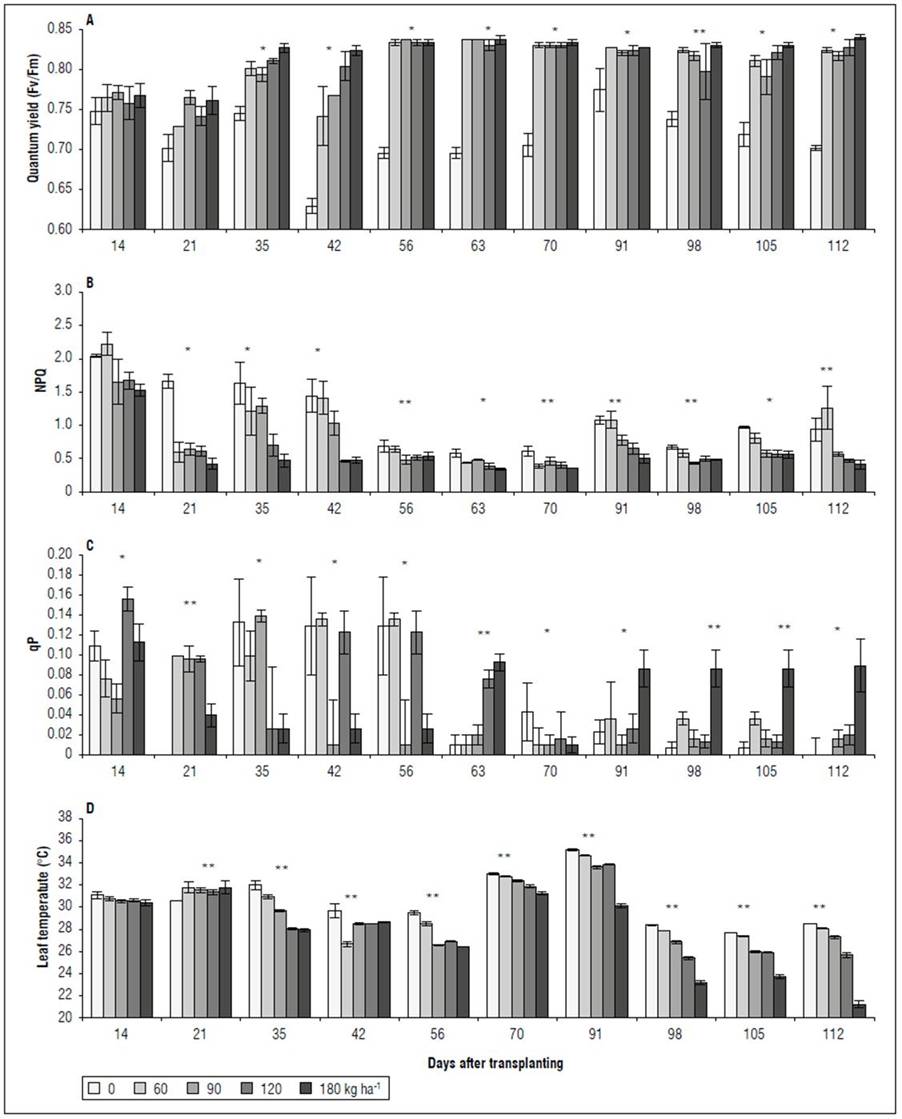
Figure 3. Effect of NPK doses on quantum yield - Qy (A), Non-photochemical quenching - NPQ (B), photochemical quenching coefficient - qP (C), and leaf temperature (D) of recently fully-expanded spearmint leaves. Bars denote mean ± standard error. Asterisks represent the difference between treatments according to Tukey’s HSD test, at 1* and 5%** probability.
Dry matter and foliar area. There were significant differences between the accumulated dry matter and the foliar area. A behavior similar to that of gas exchange and ChlF was observed. Between 14 and 21 DAT, no significant differences were found between the treatments for the variables RDM, SDM, LDM, PDM, and the total LA (Fig. 4). However, between 45 and 98 DAT, the RDM was higher in the 180 kg ha-1 treatment, significantly different from the treatments with 90, 60, and 0 kg ha-1. However, at 105 and 112 DAT, these differences did not persist, especially between the extreme doses evaluated in the 0 and 180 kg ha-1 treatments (Fig. 4A). The SDM had a behavior similar to that of RDM. Up to 63 DAT, there were no differences between the treatments; however, between 70 and 112 DAT, the 0 kg ha-1 treatment was significantly lower than the 180 kg ha-1 treatment. The treatments with 60, 90, and 120 kg ha-1 did not differ for SDM (Fig. 4B). The highest LDM was in the 180 kg ha-1 treatment at 112 DAT, higher than that of the other treatments, which did not differ significantly from each other (Fig. 4C). For LA, starting at 63 DAT and until the end of the study cycle, the response to the 0 kg ha-1 treatment was lower than that obtained with the treatments with 90, 120, and 180 kg ha-1, which did not differ significantly from the 60 kg ha-1 treatment (Fig. 5). Finally, for PDM, the trend was similar to that observed in the dry matter accumulated in the organs (RDM, SDM, LDM) independently. At 112 DAT, the 180 kg ha-1 treatment stood out over the other four treatments, presenting 45% more PDM (Fig. 4D).
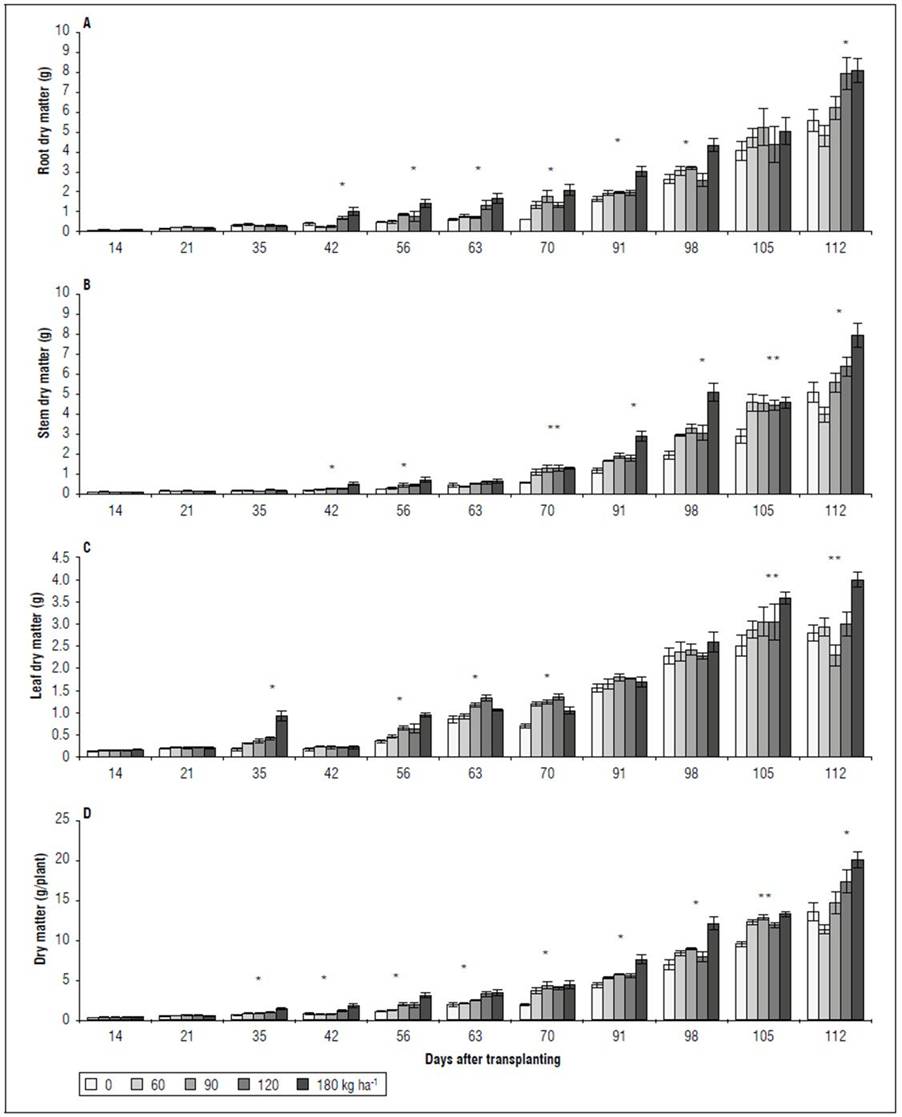
Figure 4. Effect of NPK doses on biomass partitioning in roots (A), stems (B), and leaves (C), and accumulated biomass per spearmint plant (D). Bars represent mean ± standard error. Asterisks denote the difference between treatments according to Tukey’s HSD test, at 1* and 5%** probability.
DISCUSSION
Gas exchange. The spearmint leaves presented the highest A from 35 DAT in the treatments with 120 and 160 kg ha-1, surpassing by up to 71% (105 DAT) the treatments with 60 and 90 kg ha-1 and by up to 148% (91 DAT) the treatment with 0 kg ha-1 (Fig. 2A). Hu et al. (2019) stated that N and K coordinate the diffusion and carboxylation of CO2, improving photosynthetic activity. Photosynthesis in N- and K-deficient plants is mainly limited by mesophyll conductance and RuBP carboxylation. However, research on plants with an adequate supply of N and K has shown that photosynthesis is substantially improved through a balance of regeneration and carboxylation of RuBP, favored by higher availability of N and K (Xie et al., 2020). Likewise, when modeling photosynthesis, Walker et al. (2014) showed that foliar P is decisive since, with high N levels in the leaves (3 g m-2), an increase in foliar P, from 0.05 to 0.22 g m-2, doubled the carbon assimilation rate. Results obtained by Chen et al. (2018) when evaluating growth and photosynthetic parameters in Eustoma grandiflorum (Raf.) Shinn corroborated the synergistic effect between N, P, and K, reducing the net photosynthesis in leaves with a deficiency of these three nutrients. These authors indicated that the decrease was related to a low g s ; while leaves deficient in N are also accompanied by a higher intercellular concentration of CO2 (Ci).
A reduction in stomatal opening and increased resistance are assumed to restrict g s , resulting in decreased carbon fixation and photosynthesis rates (Wang et al., 2016). The lower transpiration observed in the spearmint plants without chemical fertilization (0 kg ha-1) was associated with a lower g s (Fig. 2B) and greater resistance to water flow in the soil-plant-atmosphere continuum. Similar results were reported by Wang et al. (2016), who recorded a reduction in net photosynthesis with a low N supply in wheat (Triticum spp.) because of a decline in photosynthetic activity and low-efficiency carboxylation in the leaves. The g s was affected by serial environmental factors (availability of water and nutrients, light, CO2 levels, and temperature, among others), reducing the photosynthetic rate in C3 plants by up to 20% (Mu and Chen, 2021). Nitrogen is closely related to g s or stomatal movement. Nitrate (NO3 -), the main source of N for most plant species, regulates stomatal movements. Calcium chloride (ClCa), a NO3 -/H+ exchanger, is located in the vacuole membrane and can specifically accumulate NO3 - in the main storage compartment of the cell (De Angeli et al., 2006). On the other hand, a N deficiency promotes biosynthesis and increases the synthesis of abscisic acid (ABA), a hormone that has an essential role in the stomatal closure process (Mu et al., 2018; Mu and Chen, 2021). In addition, N-deficient plants accumulate hydrogen peroxide (H2O2), which also promotes stomatal closure (Tewari et al., 2007).
The causes of a reduced photosynthetic rate resulting from a K deficiency are attributed to a reduction in mesophyll conductance (Battie-Laclau et al., 2014; Lu et al., 2016) and g s , as well as a decrease in the concentration of chlorophyll (Martineau et al., 2017). However, Tighe-Neira et al. (2018) indicated that a K deficiency does not always limit the photosynthesis rate because a low demand in the K sink, associated with limitations in light supply and low transpiration, may not affect the fixing capacity. Pan et al. (2017) observed that the photosynthetic rate did not decrease consistently with the low availability of K in the species Brassica napus L.
Controversy exists for the main limiting effect that K has on photosynthetic activity. Oosterhuis et al. (2013) indicated that photosynthesis is limited by the opening and closing mechanisms of the stomata and, secondly, by biochemical processes. Jin et al. (2011) agreed that photosynthesis is limited by biochemical processes, such as the maximum rate of CO2 fixation by RuBisCO and the maximum rate of electron transport but also attributed it to mesophilic conductance. However, in both situations, a K deficiency has adverse effects on the metabolic phases of photosynthesis (Jin et al., 2011).
Chlorophyll fluorescence. Energy dissipation analysis is frequently used to monitor the response of the photosynthetic apparatus to environmental stress (Parkash and Singh, 2020). Stress in plants modifies the relative proportions of absorbed light energy used for quantum conversion in photosynthesis, chlorophyll fluorescence, and heat emission. Stress conditions also change the energy flow of photons through the leaf, altering its absorption, reflectance, and transmittance properties (Tremblay et al., 2012). In this study, the absence of fertilization with N, P, and K was accompanied by a significant reduction in Qy, equivalent to 14%, a significant decrease in qP and an increase in NPQ (Fig. 3).
An N deficiency can affect PSII photochemistry by decreasing PSII electron transport and Qy (Lu and Zhang, 2000; DaMatta et al., 2002). These findings are consistent with those of Du et al. (2019) for corn leaves (Zea mays L.), Huang et al. (2004) for rice (Oryza sativa L.), Wang et al. (2016) for winter wheat, and Lima et al. (1999) for beans (Phaseolus vulgaris L.), who concluded that Qy decreased significantly in the leaves of plants with a low N content.
A P deficiency generates PSII photoinhibition by reducing the efficiency of excitation energy in PSII reaction centers (Fv´/Fm´) (Xu et al., 2007). At the same time, the electron transport rate increases through PSII, and unused electrons are diverted to photorespiration, which reduces CO2 fixation (Malhotra et al., 2018). Despite the essential functions of P, Engels et al. (2012) indicated that a P deficiency had not been shown to have a direct impact on the photosynthetic electron transport chain. However, Carstensen et al. (2019) pointed out that a P deficiency reduces the rate of the enzyme ATP synthetase, which limits the output of protons to the chloroplast, acidifying the lumen of the thylakoid with consequent oxidation of hydroxy plastoquinone (PQH2) in the Cytb6 f complex, a fact that further slows the linear flow of electrons towards PSI. P is associated with the loss of chlorophyll and decreases in Qy from photo-oxidative stress (Frydenvang et al., 2015; Hernández and Munné-Bosch, 2015).
A K deficiency affects Qy by increasing thermal dissipation and reducing electron transfer efficiency, Fv´/Fm´ and qP. A reduction in the Fv´/Fm´ decreases the power and affects the Calvin cycle, with a consequent decrease in the CO2 assimilation rate. Likewise, Ye et al. (2017), who studied barley (Hordeum vulgare), indicated that plants with a K deficiency had a reduced ATPase activity, and Tighe-Neira et al. (2018) observed a decrease in the K concentration, associated with a 20% decrease in Qy, in broad beans (Vicia faba L.).
On the other hand, NPQ is an indicator of the process that dissipates the absorbed excesses of light energy as heat (Ruban, 2016) and corresponds to the dissipation mechanism of 96% of the radiation absorbed by leaves (Tremblay et al., 2012). Also, as a molecular adaptation, it represents a faster response of the thylakoid membrane to excess light (Demmig-Adams et al., 2014); this is critical since, under conditions without photoinhibition, a high and constant NPQ value is associated with a reduction in the CO2 assimilation efficiency (Murchie and Ruban, 2020). As a mechanism for dissipating excess light energy, the spearmint plants without fertilization or low doses (60 kg ha-1) presented the highest NPQ values, as compared to those that received the highest doses of compound fertilizer (> 120 kg ha-1). This is a photoprotective mechanism of the photosynthetic apparatus in the leaves of plants subjected to low NPK doses. NPQ can improve productivity by limiting photoinhibition (Hubbart et al., 2018) but can also reduce the performance of plants through its excessively protective persistence, unnecessarily reducing quantum yield under low light conditions (Kromdijk et al., 2016). As such, the 60 kg ha-1 dose saw an NPQ increase, where the Qy was not affected in the leaves (Fig. 3A). However, the leaf temperature increased by up to 7°C in the plants that received the complete fertilization plan (180 kg ha-1) (Fig. 3D).
Dry matter and foliar area. The dry matter and leaf area in the plants subjected to different NPK levels (Fig. 4 and 5) presented a behavior similar to the A results (Fig. 2). With the low NPK rates, the initial growth rates were slow, and the increase in total dry matter was not remarkable, which only became evident after 42 DAT (Fig. 4). The dry matter distribution among the plant organs was strongly affected by the NPK treatments (Fig. 4). The highest dry matter accumulation occurred in the stems and roots, less so in the leaves in all treatments. The increase was greater with the higher NPK doses. In studies that compared three spearmint cultivars, Singh et al. (2001) recorded an accumulation of dry matter that varied between 7.07 and 9.18 g/plant, values lower than those of this study. The doses higher than 120 kg ha-1 saw an accumulation greater than 14.20 g/plant, distributed in the leaves (25%), stems (35%) and roots (40%).
The leaf area decreased as the NPK doses were reduced (Fig. 5). Zhao et al. (2016) highlighted the importance of N by confirming that a deficiency of the element in sorghum plants (Sorghum spp.) led to a reduction in leaf area, chlorophyll content, and photosynthesis, which resulted in a decrease in leaf area with a low accumulation of dry matter. Similar results were obtained by Roveda-Hoyos and Moreno-Fonseca (2019) when evaluating P deficiencies in cape gooseberry (Physalis peruviana L.) plants. Qiu and Israel (1994) pointed out that a reduction in leaf area expansion and a consequent decrease in biomass from a P deficiency is more important than the effect of P deficiencies on the photosynthetic rate per unit of the leaf area. On the other hand, Gerardeaux et al. (2009) showed that an early K deficiency in cotton plants (Gossypium ssp.) restricts the growth of the leaf area, where the number and individual size of the leaves are smaller.
According to Timlin et al. (2017), a smaller leaf area is associated with an accelerated decrease in the number of leaves because of faster senescence when the plants are subjected to a low supply of NPK, especially P. The growth and expansion of leaves involve two main processes: expansive growth and structural growth, which depend on water to increase cell volume and carbon and nutrients for structural growth (Pantin et al., 2011). Since leaves are essential for photosynthesis, NPK is indispensable to building initial infrastructure. Nitrogen, P, or K deficiencies suppress growth, accelerate senescence, and decrease chloroplastic pigment concentrations in leaves (Tewari et al., 2007).
As in the gas exchange variables, the synergistic effects between nutrients are recognized. Schlüter et al. (2013) indicated that P stress affects N dynamics, where the absorption of N is down-regulated under conditions with a P deficiency. At the same time, Hou et al. (2018) emphasized the importance of the favorable effect of the correlation between N and K on photosynthesis.
The nutritional management of spearmint is essential for the development of biomass; between 250 and 300 kg ha-1 of N, 55 and 110 kg ha-1 of P2O5, and about 375 kg ha-1 of K2O are required to obtain better yields (Brown et al., 2003). These values are much higher than those used in Colombia for target fertilization, where 18 kg of N, 54 kgof P, and 18 kg of K are used in 1 ha, with a yield of 10-12 t ha-1 of fresh leaves (Agronet, 2021), less than the 15 to 20 t ha-1 of fresh leaves seen worldwide (Wikifarmer, 2021).
CONCLUSION
Increasing the NPK doses favored the photosynthetic performance and quantum yield in the spearmint plants. The highest NPQ values were recorded with the lowest NPK doses. High NPK doses increased the accumulated dry matter and the foliar area, contributing to a higher yield. For the gas exchange variables, in general, the doses of 120 and 180 produced the best rates of photosynthesis in the Mentha plants. A similar trend was observed for the accumulated dry matter and leaf area, parameters associated with yield.