INTRODUCTION
Fruit trees belong to plant species that are more affected by environmental stresses, and this vulnerability is becoming increasingly dangerous because of climate change, where regional climate regimes are unpredictable (Rachappanavar et al., 2022). Climate change harms many fruit crops in terms of normal growth and development, such as deficient pollination, reduced fruit set and panicle emergence, sunburning, low color development and sugar content and delayed fruit maturation, along with poor quality that decreases yield and productivity of orchards (Chawla et al., 2021).
Many fruit-growing regions have stress events because of drought, salinity, high incidence of solar radiation (PAR and UV) and excessive heat load (Brito et al., 2019), constituting the four types of stress with the most limiting factors for agricultural productivity at a global level, with enormous synergistic effects on fruit growth, production and quality. In the case of sunburn on fruits caused by high temperatures and intense sun radiation, Park et al. (2022) considered physiopathies that will increase because of global warming.
Schrader (2011) recognized sunburning an important type of solar radiation injury with huge economic losses in fruits, vegetables and ornamental plants. Sunburn on fruits arises from high solar radiation (e.g. at high altitudes) and high air temperature combined with low relative humidity (RH), as well as when there are very few leaves to protect recently set fruits (Baiea et al., 2018). Authors such as Restrepo-Díaz and Sánchez-Reinoso (2020) pointed out that thermal stress in combination with excessive solar radiation generates physiological disorders in fruits, such as sunburn, that reduce commercial value.
High solar radiation that causes solar injuries exceeds the natural protection of fruit trees, generating large economic losses in crops, especially in arid and semi-arid regions (Schrader, 2011). There are other factors such as wind speed, RH, crop management practices, and fruit acclimatization that indirectly influence the origin of sunburn and that, by themselves, cannot cause this damage but interact with direct factors in the development of symptoms (Racsko and Schrader, 2012). According the second author's observations, damage is more frequent in fruits exposed to the west, where they receive radiation perpendicularly, when the atmosphere and plants have lost humidity, with the most severe damage in the dry seasons in crops that are sensitive to excess radiation and temperature with few leaves in that part of the plant.
From 2010 to 2019, the days with a risk of sunburn for fruits more than doubled from previous decades, therefore damage is expected to increase with global warming (Park et al., 2022).
Fruit sunburn by photo-oxidative stress is irreversible (Bolivar-Medina and Kalcsits, 2022), and fruit losses vary depending on species, variety and season of the year, affecting between 6 and 30% of production (Schrader et al., 2008; Tsai et al., 2013), especially in regions with warmer climates at low latitudes (Racskó et al., 2010).
In this context, it should not be forgotten that solar radiation is the most important source of energy for photosynthesis and thus ensures life on Earth (Kochhar and Gujral, 2020). Thus, and up to a certain level, solar radiation improves the production and quality of fruits (Musacchi and Serra, 2018) and can reduce the impact of diseases and pests on crops (Lal and Sahu, 2017). Woolf and Ferguson (2000) characterized sunburning as a result of excess, but possibly beneficial, solar radiation in fruits.
There is a lot of information on the effect of excessive solar radiation on deciduous fruit trees, especially apple (Racsko and Schrader, 2012), and scarce information on fruit trees originating from subtropical and tropical zones although there are several studies, mainly for of citrus (e.g. Rodriguez et al., 2019; Park et al., 2022). In the tropics, excess radiation and high temperatures, in many cases, are the factors that most limit crop growth and production (Wahid et al., 2007).
Sunburn damage can occur any year and in all regions of the world, generating large losses in many fruit crops (Racsko and Schrader, 2012). In areas with a high incidence of fruit sunburn, growers must apply management practices that reduce this physiological disorder, such as the use of tolerant species and varieties, appropriate crop management such as pruning and plant training, efficient irrigation, soil cover plants and intercropping, shading nets, suppressors (kaolin or calcium carbonate), fruit bagging and chemical protectants, among others (Lal and Sahu, 2017).
Climate change is projected to affect tropical and subtropical regions the most, where temperatures are already higher initially, and increased heat, UV radiation, and reduced water availability can greatly affect crop yields at these low latitudes (Rehman et al., 2015; Yohannes, 2016). This review explores how these conditions affect the fruits of species, mainly from tropical and subtropical origin, as a result of sunburn, and possible management alternatives for their mitigation.
CLIMATE FACTORS CAUSING SUNBURN
In regions with high temperatures, sun damage is the first cause of fruit loss (Manja and Aoun, 2019). Sunburn is caused by high solar radiation and the combination of high temperature with low RH (Baiea et al., 2018). In general, an increase in the environmental temperature over 32°C increases the risk of solar damage, requiring the implementation of mitigation plans (Bolivar-Medina and Kalcsits, 2022).
As a result of the stratospheric ozone layer depletion, the amount of UV-B radiation (280-320 nm) reaching the planet's surface is increasing (Abd-Allah et al., 2013). This situation is aggravated in tropical conditions that suffer from higher levels of UV light than temperate zones because of the small solar zenith angle and the thinner ozone layer in the stratosphere (Krause et al., 1999).
Solar radiation (visible, UV and infrared) increases at high altitudes due to the reduced atmospheric layer that filters the sun's rays (Fischer et al., 2022a). UV radiation increases with elevation, as reported by Benavides et al. (2017) in Colombia. According to latter authors, UV radiation increases 10-12% with each increase of 1,000 m in altitude. Fruit production in Colombia occurs up to 3,200 m above sea level (a.s.l.), with reports of sunburn at high altitudes in species such as avocado, pineapple, citrus, passionfruits and feijoa, among others (Fischeret al., 2022a). According to Guerrero (2014), solar radiation consists of 50% infrared (IR) light, 45% visible light and 4-7% UV light, with IR and visible lights being the main causes for fruit heating, while UV causes fruit browning.
The number of sunny hours with high temperatures during fruit ripening greatly influences the damage; in addition, sunburning can occur when cold or mild weather ends abruptly followed by hot and sunny weather (Lal and Sahu, 2017). Likewise, periods with a decrease in heat can be beneficial in hot climates because they promote the photosynthetic rate during the hottest hours of the day and thus protect against sunburn (Kalcsits et al., 2017; Manja and Aoun, 2019).
According to Woolf and Ferguson (2000), high light intensity, heat and water stress increase the propensity for sunburn, causing sun injuries as the result of high temperatures on the fruit skin. These conditions occur in crops in arid and semi-arid regions, where global warming, excessive solar radiation and water scarcity are the most aggravating environmental restrictions (Shaban et al., 2021). Several authors, cited by Tsai et al. (2013), pointed out that the surface temperature of fruits grown in subtropical and tropical areas can reach up to 40°C and can be even higher when exposed to direct sunlight.
Factors, such as RH and wind speed, influence the effect of solar radiation and temperature on the surface of fruits, modifying the severity of symptoms, but these weather components cannot alone cause the damage (Racsko and Schrader, 2012). As wind speed increases, the temperature of fruits decreases, as observed by Thorpe (1974) in apple trees, in which an increase in speed from 0.3 to 4 m s-1 reduced the surface temperature of fruits by 5°C. According to the second author's observations, the incidence and severity of damage are influenced by the relationship of unfavorable weather conditions (temperature, RH and day length) that interact with the edaphic characteristics of soil water retention (related to texture, structure and depth), along with morphological (such as depth and vigor of the roots) and physiological characteristics (such as hydraulic conductivity) of plants.
RESPONSE OF FRUITS TO SUNBURN
According to Blanke and Lenz (1989), fruits suffer more than leaves from sunburn as a result of less efficient mechanisms to use or dissipate excessive solar radiation that increases fruit temperature. Parchomchuk and Meheriuk (1996) observed a fruit surface temperature 10-15°C higher than air temperature in 'Jonagold' apples. Sunburned fruits show discoloration and varying degrees of cell death (Mishra et al., 2016).
Racsko and Schrader (2012) emphasized that, for the three types of sunburn, photooxidative sunburn, sunburn browning and sunburn necrosis, the direct, inducing factors are radiant heating and/or exposure to excessive sunlight. These three different types of sunburn were described by Muñoz and Munné-Bosch (2018) and Musacchi and Serra (2018): photooxidative sunburn, where fruits become photobleached by excessive amounts of light in the canopy; sunburn browning, caused by increases in ambient light and temperatures (46-49.8°C) that are related to a serious deterioration of chlorophylls and carotenoids, resulting in a bronze-brown-yellowish coloration; and sunburn necrosis, the most serious damaging, resulting from even higher temperatures, destroying tissues exposed to direct sunlight and causing a loss of cellular integrity and cell leakage (Schrader, 2011; Racsko and Schrader, 2012). Sunburn necrosis in apples occurs when the fruit surface temperature reaches 52±1°C for 10 min, causing cell death (Schrader et al., 2008). Very dry environment, especially with high temperatures, promote sunburn (Baiea et al., 2018).
According to Parkhe et al. (2022), high solar radiation combined with high temperature generates the formation reactive oxygen species (ROS) in plant tissues (Parkhe et al., 2022). These authors stated that ROS induce a loss of membrane integrity, leading to electrolyte leakage that affects cell proteins and lipids, ultimately causing cell death. In addition, a serious sunburn affects the cuticle and damages not only epidermal tissue but also subepidermal tissue. Tsai et al. (2013) highlighted that sunburn also disturbs the photosynthetic systems and destroys the oil glands, leading to a loss of water and a decrease in growth and yield of fruit trees. Moutinho-Pereira et al. (2009) reported that physiological processes such as rates of transpiration, respiration and photorespiration are altered by a high photosynthetic photon flux density (PPFD), which also causes photoinhibition and chlorosis in plants (Casierra-Posada, 2007).
During fruit development, sunburn causes skin discoloration and a high respiration rate that degrades the chlorophyll and anthocyanins (Merzlyak et al., 2008). Solar radiation is important for photosynthesis, but an excess generates light saturation and a reduction in the efficiency of radiation use by plants (Taiz et al., 2017). High light intensities and temperatures damage photosystem II (PSII), and excessive temperatures decrease the photochemical activity and the primary light energy conversion efficiency (Fv/Fm) of PSII (Sun et al., 2018). Damage can be quantified using chlorophyll fluorescence (Sánchez-Reinoso et al., 2019).
In apple fruits injured by sunburn, the activities of superoxide dismutase (SOD) and catalase (CAT) were increased to counteract the overproduction of ROS (Zhang et al., 2015). CAT catalyzes the degradation of hydrogen peroxide, and its performance is largely dependent on metabolic activity and resistance to oxidative stress or diseases (Bagheri et al., 2021).
Carotenoids, anthocyanins and phenols are also important in eliminating the overload of hydrogen peroxide generated under conditions of environmental stress (Hinojosa-Gómez et al., 2020). Carotenoids transfer the energy of absorbed light in a molecular form that does not participate in the simple transfer of absorbed light but prevents destructive photo-oxidation (Felicetti and Schrader, 2009). According to Zhang et al. (2015) and Muñoz and Munné-Bosch (2018), the protection against sunburn occurs through the activation of xanthophyll cycle-dependent excess energy dissipation system, through low molecular weight antioxidants (carotenoids, glutathione, tocopherols, ascorbate and flavonoids) and through the efficient use of the ascorbate-glutathione cycle, along with other enzymatic antioxidants.
Prolines also contribute to the tolerance of plants under stress conditions by establishing a protective layer formed by water molecules that maintain cellular homeostasis (Yin et al., 2013). High levels of UV-B radiation cause harmful effects at the physiological, biochemical and morphological tissue levels (Brito et al., 2019). Phenolic compounds have a strong absorption of UV radiation between 200 and 300 nm, protecting fruits from harmful radiation (David and Felicetti, 2008).
SOME EFFECTS ON FRUIT QUALITY
For growers, fruit quality is decisive to ensuring economic return for fruit-growing activity and is increasingly a relevant factor in production (Padrón-Mederos et al., 2020). As pointed out by Munné-Bosch and Vincent (2019), the exposure of fruits to excess radiation can deteriorate quality, organoleptic characteristics and consumer acceptance.
The main symptoms of sunburn are the different types of discoloration of the fruit's skin, which can vary, with the development of whitish or yellowish spots on the fruit (Manja and Aoun, 2019). For example, in the case of citrus fruits, symptoms range from a light discoloration or yellowing to spots or necrotic areas (Mishra et al., 2016; Rodriguez et al., 2019), while on the rough skin of avocado Hass fruits exhibit a slight yellowing with reddish colorations or rounded dark brown lesions (Ramírez-Gil and Morales, 2019).
The physiological disorder caused by exposure to excessive solar radiation seriously decreases fruit quality, depending on sunburn types, which can cause necrosis, browning, and photooxidative discoloration (Racsko and Schrader, 2012). The occurrence of high temperatures and droughts during periods of high radiation at the stage of fruit maturation might cause discoloration of the epidermis, delay maturity and increase cracking and creasing, which greatly affect crop yield (Muchie and Assefa, 2021). In addition, the adverse effect of excessive temperatures that limit the production of auxins in fruits leads to the development of smaller fruits (Wahid et al., 2007).
Excessive and prolonged solar radiation not only induce sunburn in fleshy fruits but also increase the temperature of affected epidermal cells, causing fruits cracking and pathogens infection through these unprotected tissues, increasing fruit loss (Fischer et al., 2021a). Woolf and Ferguson (2000) reported in several varieties of avocado, including 'Hass', that in fruit developed in direct sunlight the pulp temperature reached around 40°C. Such fruits showed a greater tolerance to stress caused by high and low temperatures during postharvest handling.
SUNBURN MITIGATION STRATEGIES
Fleshy fruits are high susceptible to sunburn given their lack of sufficient resistance mechanisms. Therefore, growers are required to select cultivars tolerant to sunburn (Narayan and Sahu, 2017), and adopt a series of different measures to control this physiopathy (Baiea et al., 2018). The mitigation of the impact of high temperatures is essential in the orchards to guarantee fruit productivity and quality, given the increase in temperature caused by climate change and variability in recent years (Kalcsits et al., 2017). Therefore, the implementation of protected cultivation practices in fruit crops is recommended, applying sustainable and environmentally friendly management to reduce crop damage (Manja and Aoun, 2019).
Racsko and Schrader (2012) classified strategies to mitigate the adverse effects of sunburn into (1) climate-improving techniques, (2) suppressants, and (3) chemical protection. Musacchi and Serra (2018) stated that the first group of strategies includes the use of shade nets, fruit bags and evaporative cooling, while the second includes particle films (as e.g. kaolin clay). In the third strategy, trees can be treated with tocopherol, abscisic acid (ABA), ascorbic acid and/or anti-transpirants, where anti-transpirants, with their effect on reducing water loss through transpiration, increase the temperature of leaves and young fruits (Racsko and Schrader, 2012).
The practices to improve the microclimate of plantations and fruit conditions, include pruning and training plants, leaving a sufficient leaf area above the fruits, along with intercropping, efficient irrigation, and use of over tree sprinklers (Narayan and Sahu, 2017; Muñoz and Munné-Bosch, 2018). In addition, cultural practices should also include removal of burnt and/or cracked fruits at the beginning of the growing season, since they do not have a market value (Stuckens et al., 2011) and can cause phytosanitary problems.
Shade netting reduces the adverse effects of incoming solar radiation to the plantation by reducing the temperature and controlling sunburn (Racsko and Schrader, 2012; Mditshwa et al., 2019; Mukherjee et al., 2019). In apple crops in southern Brazil and in Argentina, shade netting has been used mainly to prevent damage caused by hailstorms, also proving efficient to reduce sunburn to the fruit (Amarante et al., 2011; Raffo et al., 2015). The light transmittance for these shading nets is between 20 and 70% (Manja and Aoun, 2019).
The shading factor of the nets refers to their ability to absorb and reflect radiation in the visible spectrum (Castellano et al., 2008). This characteristic is affected by color, where black, blue, green, red, yellow, white, gray and transparent nets are used for different purposes (Castellano et al., 2008). Possibly, the effect of net on sunburn reduction depends on cultivar, as reported by Amaranteet al. (2011) in apple trees, in which the white shade net reduced the incidence of sunburn in 'Gala' but not in 'Fuji'.
Shade nets in apple orchards reduce fruit sunburn as a result of shading, reduced temperature, increased RH, and increased water-use efficiency of the plants (Amarante et al., 2012). Shade nets reduce the daytime temperature on hot days through the interception of solar radiation or the shadow effect, which is greater than the temperature gain caused by the reduction of air circulation or the greenhouse effect. Covering an orchard with a shade net reduces wind speed and increases RH in the growing environment, thus reducing transpiration from the leaves. The higher water content resulting of lower transpiration, combined with a lower heating of the fruits due to the reduction of radiation reduces sunburn in apples (Amarante et al., 2011).
However, according to Lee et al. (2015), depending on the net type, a level of scattered radiation below the screen can still generate this physiological disorder in fruits. These authors reported fruit sunburn even in Ponkan mandarin under a white shading net (20%).
The change in the microclimate caused by shading nets influence the physiology of fruit trees (Mupambi et al., 2018), increase the size and quality of the fruits (Basile et al., 2012), increase the leaf area (Shaban et al., 2021) and can reduce the entry of insects into the plantation as a result of their color preferences (Ben-Yakir et al., 2008). The color of net shading material also influences fruit quality throughout responses, mediated by phytochromes (red and far-red receptors), cryptochromes, and phototropins (blue light receptors), involving upregulation of specific genes, e.g. those responsible for the synthesis of phenolic compounds during fruit development (Tinyane et al., 2018).
Fruit bagging protects against excess solar radiation and improves quality and color of the fruit (Feng et al., 2022). For this technique, the bags can be manufactured with different materials (such as paper, fabric and plastic) and of different colors. However, the removal of the bag during high solar radiation can cause sunburn (Feng et al., 2022). Therefore, fruits that need exposure to light (UV) to stimulate color development during ripening, e.g. in type II red apples, bag must be removed before this stage (Ali et al., 2021). The use of bags represents a physical barrier around the fruit, protecting them from high solar radiation, as well as against pathogens and environmental stresses (such as high temperatures, rain, hail, wetting and air movement (Sharma et al., 2014).
The application of suppressants such as kaolin, a clay mineral which occurs naturally (Mohamed et al., 2019), reduce abiotic stress in fruit trees by forming a film of protective white particles on the surface of leaves, stems and fruits that increase the reflection of high radiation and, therefore, decreases temperature and damage from sunburn (Glenn, 2012; Glenn and Puterka, 2005). Particle film technology represents a joint knowledge on mineral technology, light physics and insect behaviour, comprising studies on insect-pest control and plant physiology.
Kaolin limits the loss of transpired water and maintains a rather high stomatal conductance, improving the water status in plants (Brito et al., 2019). The white kaolin mineral (Al2Si2O5(OH)4) is chemically inert, non-toxic, non-abrasive and easily dispersible in water (Brito et al., 2019). Kaolin clay is difficult to remove completely from fruits after harvest and, therefore, it appears to be pesticide residues (Araújo and Britto, 2021). There are other films based on kaolin particles, such as the highly processed Surround®, which is more effective in reflecting UV radiation than unprocessed kaolin or calcium carbonate (Glenn and Puterka, 2005).
Researchers agree that only the selection of adapted crops and varieties and the adoption of mitigation management are sustainable to control sunburn of fruit trees under this climatic stress (Lal and Sahu, 2017; Goraet al., 2019; Chawla et al., 2021). The minimum temperature of the fruit surface to induce solar injuries is modified according to the variety of the fruit species (Schrader, 2011). On the other hand, there is little information on the molecular mechanisms of plants acclimatization to a combination of two different stresses (Mittler, 2006), as is the case of excessive radiation and temperature occurring at the same time. Also, Rehmann et al. (2015) pointed out that many plant breeding programs directly or indirectly include selection for stress tolerance, and average yields continue to be below plants genetic potential because the occurrence of various field stresses that limit yields.
According to Rachappanavar et al. (2022), one of the promising strategies to alleviate abiotic stress includes plant breeding techniques with the use of different phytohormones, especially gibberellic acid, abscisic acid, auxin, brassinosteroids, salicylic acid and jasmonates. These phytohormones trigger cascades of specific signals on abiotic perception and fluctuations in key levels of these hormones and crosstalk between them occurs as an early response to stress. Rachappanavar et al. (2022) argued that a higher degree of tolerance to environmental stress at the molecular level through the adjustment of growth factors plays a key role in plant survival.
SOME EFFECTS OF SUNBURN ON DISEASES AND PESTS
The epidermis of fleshy fruits affected by sunburn with susceptibility to cracking are more prone to attacks by pathogens (Fischer et al., 2016; 2021a) and develop postharvest disorders mainly because of the incidence of fungal diseases (Lal and Sahu, 2017).
For papaya, Reyes (2012) reported that the bunchy top (induced by phytoplasma), especially in var. Hawaiana, causes the loss of foliage, leading to fruit sunburn, where the entire plant dies, and growers cover fruits with newspaper to protect them from sun damage.
The use of shading nets to protect fruits from sun exposure, in many cases, also better protect against anthracnose, as in the case of the Hass avocado protected with a red net (Tinyane et al., 2018). In a Keitt mango crop, a 60% shade protected better against powdery mildew and malformed panicles (Shaban et al., 2021).
EXAMPLES IN DIFFERENT TROPICAL AND SUBTROPICAL FRUITS
The severity of sunburn damage to the fruits depends on several factors, mainly the aforementioned weather conditions, but also the variety, nutrition, hormones and soil moisture (Baiea et al., 2018), in addition to the period of fruit development and harvest (Lopes et al., 2014).
Avocado
Young fruit trees must be protected against high radiation by shading or by painting white the trunk. In addition, great care must be taken in pruning in areas of high radiation since avocados are especially susceptible to these stressful conditions (Kourgialas and Dokou, 2021). Depending on the season, in the summer of the subtropics and also during the dry seasons in the tropics, the incidence of sunburn (Fig. 1) increases, as is the case in Michoacan (Mexico), where avocados exported in October had 26.4% sunburn, but only 8.1% in April (Herrera et al., 2017).
Ramírez-Gil and Morales (2019) observed in the 'Hass' avocado trees with poor foliar coverage and exposed to direct sunlight, that the fruits presented a slight yellowing with reddish colorations or rounded dark brown lesions; meanwhile, under these conditions, green-yellow colorations were detected in the stems, turning brown to black. In a study to reproduce these symptoms, Ramírez-Gil and Morales (2019) observed that the symptoms in the fruit and stems appeared after 19.5 and 62.4 d at full sun light exposure, respectively.
Covering 'Hass' with white or blue shading nets decreased the temperature in the canopy of the trees (Tab. 1) and fruit sunburn was reduced to 10% (Tinyane et al., 2018). Blakey et al. (2016) reported reduced incidence of sunburn in 'Gem®' and 'Carmen®-Hass' avocado trees covered with white shade nets on the top (20% shading) and green nets on the sides (40%) or crystal shade nets (30%), placed on the top at a height of 6 m.
According to Wolstenholme (2007), avocado trees require 2,000 h of direct sunlight per year, being important to guarantee a layer of leaves to protect the fruits and paint trees structures exposed to high solar radiation such as the branches and trunk, with lime paint. Aside from strict protection of the canopy from direct solar radiation, Schaffer and Whiley (2003) recommend a combination of techniques such as plant spacing, pruning and tree thinning, to maximize the interception and use of light by avocado trees, avoiding excessive shade, so that a high percentage of leaves within the canopy are above the light saturation point for photosynthesis.
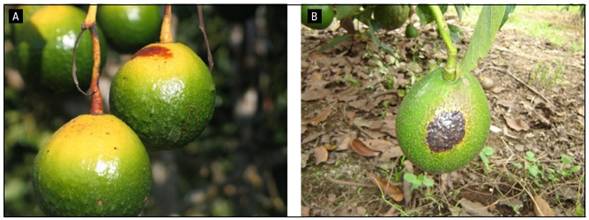
Photos: J.O. Orduz-Rodríguez (A) and G. Fischer (B).
Figure 1. Yellowing with reddish coloration (A) or rounded dark brown injuries (B) on avocado fruits.
Table 1. Examples of the effect of sunburn on tropical and subtropical fruits including mitigation treatments.
Species, variety | Treatment | Increase | Decrease | Author(s) |
---|---|---|---|---|
Avocado (Persea americana), var. Hass | Trees covered with blue, red or white shading nets with 20% shading | Up to 40% of non-marketable fruits with sunburn on uncovered trees; increases of oleic acid and incidence of anthracnose in covered trees | White and blue shading nets reduce the canopy temperature by 7.6 and 7.3°C, resp., with 10% sunburn; less anthracnose under red shade nets | Tinyane et al. (2018) |
Citrus Mandarin (Citrus unshiu), var.Okitsu | Kaolin 95% in doses of 2 and 4% applied 2, 3 or 4 times | The applications of kaolin had 92 and 98% fruits without sun injuries compared to 74% of the control; higher content of TSS and TTA at the doses of 4% and 4 applications | 4 applications at 2% or 3 applications at 4% kaolin decreased sunburn by 3% | Chabbal et al. (2014) |
Citrus Satsuma mandarin (Citrus unshiu), var. Nichinan 1 gou | Comparison of severity of sunburn damage using 4 grades: I slight, II mild, III moderate severe, IV severe | Increased damage to epidermis and oil glands with severity (I to IV); total polyphenols and antioxidant activity increased in fruits without sunburn and those of grade III; higher firmness in sunburned fruits | Chlorophyll (a, b and total) decreased in the skin and carotenoids diminished in the pulp with increasing sunburn severity; TSS and TTA decreased in sunburned fruits | Kim et al. (2022) |
Citrus Mandarin (Citrus reticulata), var. Nagpur | 2 applications of (A) kaolin 3% and (B) 5%, (C) MgCO3 3% and (D) 5%, (E) 15 ppm GA3+1% urea, (F) (15 ppm GA3+1% urea) and 3% kaolin, (G) (15 ppm GA3+1% urea) and 5% kaolin, (H) (15 ppm GA3+1% urea) and 3% MgCO3, (I) (15 ppm GA3+1% urea) and 5% MgCO3, (J) control | Control (untreated) fruits had up to 9.0% increase in sunburn; sun injuries increased to 4.2% in the southwestern part of the tree compared to 0.7% in the northeastern direction | Lower incidence of sunburn on fruits of treatments G (3.2%) and I (3.3%). Lower incidence of sunburn (1.6%) in the southwestern position of the tree for treatments I, G and B; reduction of sunburn in treatments with 5% kaolin or 5% MgCO3 | Parkhe et al. (2022) |
Citrus Murcott mandarin (Citrus reticulata × Citrus sinensis) | (A) fruit bagging with white paper bags, (B) application of CaCO3; (C) white shade net (20% shade), (D) green shade nets (30% shade) and (E) black shade nets (50% shade) | Increment of 15 g for fruit fresh weight under the black shade net | No sunburn for treatments A, C, D, E; CaCO3 with 4.4% sunburns; shading nets reduced canopy temperature by 4-5°C | Tsai et al. (2013) |
Citrus Grapefruit (Citrus × paradisi), var. Rio Red | In the first year, in August, reflective solutions (R, based on Ca) were sprayed (1) at 9.35 L ha-1 and (2) 18.70 L ha-1; and an anti-transpirant (AT, based on methen/pinolene) was sprayed at 14 L ha-1; in the second year, in May, June and July, R+AT were sprayed | The anti-transpirant (AT) increased the temperature of fruit and leaf by about 0.83 and 0.2°C, resp.; trees treated with AT had more fruits with sunburn in the first (6%) and second (8.2%) years | The R reduced the temperature of fruits and leaves by 0.2°C; R 1 and R 2 reduced stomatal conductance by 1.3 and 3.3%, resp.; AT reduced stomatal conductance by 8.3%; treatments with R reduced sunburn by 4.9% and R+AT reduced sunburn by 1.8%, compared to the control | Rodriguez et al. (2019) |
Mango (Mangifera indica), var. Keitt | Application of kaolin at 25, 50 and 75 g L-1, and Screen DuoTM at 6, 12 and 18 cm3 L-1, one or two applications | 12 and 18 cm3 L-1 of Screen DuoTM improved fruit quality (TSS, vitamin C, and firmness) | Screen DuoTM at 12 and 18 cm3 L-1 reduced sunburn; kaolin and Screen DuoTM reduced TTA | Baiea et al. (2018) |
Mango (Mangifera indica), var. Keitt | Trees covered with white shade nets providing 60, 40 and 0% shading and 100, 85 and 70% of the irrigation level | The higher level of shading increased leaf area and fruit set; 40% shading and 70% irrigation increased yield, with 0% sunburn | The increase of shading reduced sunburn, and chlorophyll and proline contents | Shaban et al. (2021) |
Pineapple (Ananas comosus), var. Golden (MD-2) | Blue shade nets (70% light transmittance) placed at 0, 20 and 40 cm above the plant, 30, 45 and 60 d after flower fading | Better fruit quality (size, firmness, pulp yield, TSS) provided by net placed 0 cm above plants, 30 d after flower fading | Plant covering 30 d after flower fading had the most substantial reduction of sunburn; fruit had the lowest TTA for net placed at 0 cm above the plant 30 d after flower fading | Zhao et al. (2020) |
Pineapple (Ananas comosus), var. Perola | Fruit treatment with nanoparticles of calcium carbonate (NCC) at 0, 12.5; 25; 50; 100 and 200 g ha-1 | NCC applications improved the visual characteristics of the rind and pulp, and increased firmness, TSS, TSS/TTA ratio and ascorbic acid content | NCC reduced sunburn, yellowing index, heating and TTA content of the fruit | Teixeira et al. (2022) |
Pitaya | Plants covered with nets providing shades of 25% (white shading net), 50% (black shading net) and 75% (black shading net) | Shading increased chlorophylls content (a, b, and total) and the green color of the cladodes; 50% shade increased fruit fresh weight | Shading nets reduced sunburn and TSS; 50% shade reduced fruit cracking | Chang et al. (2016) |
TSS: total soluble solids; TTA: total titratable acidity; resp.: respective
Banana
According to Rachappanavar et al. (2022), the critical temperature for sunburn in banana is above 38°C, which causes chlorophyll degradation and stops plant development. Banana bunch bagging is commonly used to reduce blemishes and improve fruit skin coloration, as well as to reduce the incidence of sunburn, pests and diseases, agrochemical residues, mechanical damage and damage by birds (Santosh et al., 2017; Martins et al., 2019).
Lima et al. (2020), by comparing polyethylene (PE) bags with black (with thickness of 6, 8 and 10 μ) and blue (3 μ which is the most commonly used in banana) colors for bunches protection in the Nanica variety in the São Paulo State (Brazil), reported sun injuries on hot days, especially at the end of winter, for bunches protected with black bags. Muchui et al. (2010) studied perforated PE bags, with opaque blue and bright blue colors in 'Williams' banana, bagged when the hands had started to curve upwards. Bagged fruits had a better appearance and were cleaner, and fruits from higher hands exhibited sunscald, especially in opaque blue bags, that increased the temperature inside the bag on hot days. To avoid this damage, Muchui et al. (2010) recommended keeping enough leaves above the bunch and the use of blue bags with a reflective cover.
Citrus
Most research on sunburn damage and its mitigation in tropical and subtropical fruit trees has been conducted on citrus (Fig. 2). As in many other species Citrus are exposed to different abiotic stress (such as excessive light, ultraviolet radiation, heat, freezing, flooding, salinity, and drought) limiting plant growth, fruit production and quality (Rao et al., 2022). In laboratory and field studies, Kimet al. (2022) reported rind damage of Satsuma mandarin exposed at temperatures exceeding 47°C. In the same variety, Park et al. (2022) reported sun injuries when the temperature rose above 46°C for 3 hours or more.
In Taiwan, Tsai et al. (2013) reported that the average sunburn damage on Murcott mandarin fruit was 13.6% when no control measures were applied, especially on early varieties.
In citrus fruits, sunburn destroys the oil glands of the flavedo, and the damage can extend to the underlying cells (Malik et al., 2021). The main symptom of sunburn is discoloration of the fruit skin, which can range from light discoloration or yellowing to spots or necrotic areas (Mishra et al., 2016; Rodriguez et al., 2019). Park et al. (2022) pointed out that susceptibility of citrus varieties to sunburn and its impact on marketability depends on fruit characteristics, such as flavedo thickness (Rodriguez et al., 2019).
In Tahiti limes, frequent damage in Colombia includes sunburn or sunspots, occurring in fruit on the tree or in fruit exposed to direct sun in the orchard or during transportation, which limits fruit export (Botina et al., 2019). Symptoms in lemon fruit are yellow or pale green tones (resulting from pigment changes in the fruit skin) that in extreme cases can give rise to necrotic areas of a dry and hard appearance (Chabbal et al., 2014).
Applications of kaolin at doses of 3 to 5%, twice or four times during fruit development, reduced sunburn in mandarins (Tab. 1) (Chabbal et al., 2014; Ennab et al., 2017; Parkhe et al., 2022). Mohamed et al. (2019) found the best control by treating trees with 4% kaolin + chelated copper at 2.5 cm3 L-1. Mohsen and Ibrahim (2021) reported best results in reducing sunburn and cracking and improving quality of Murcott mandarins by treating trees with 2% kaolin + 0.3% potassium silicate, applying it every 3 weeks from mid-May to mid-August (in the N hemisphere).
In Murcott mandarins, fruit bagging with white paper bags and orchard protection with green (30% shade) or black (50% shade) shading nets were equally effective to control fruit sunburn (Tsai et al., 2013). On citrus, applications of kaolin were more effective to reduce fruit sunburn than CaCO3 (Tsai et al., 2013; Mohamed et al., 2019).
Mango
In China, in anticipation of global warming, mango is now being grown at higher altitudes (Zhang et al., 2022), where incidences of sunburn occur because of high solar radiation (Fischer et al., 2022a). In fruits such as mango, Fischer and Orduz-Rodríguez (2012) observed that prolonged exposition to high solar radiation can cause thermal degradation of anthocyanins in the fruit peel, turning it unnaturally bluish and black.
The Keitt variety has a low canopy with a lower number of protective leaves, which increases fruit sunburn when exposed to higher solar radiation (Hamdy et al., 2022). This variety treated with 6% kaolin had reduced fruit sunburn, associated to the increase of leaf area, canopy volume, leaf carotenoids, photosynthetic pigments (chlorophylls a and b) and yield (Hamdy et al., 2022). Baiea et al. (2018) reported in 'Keitt' mango that Screen DuoTM application was more efficient to reduce fruit sunburn than kaolin (Tab. 1). Also, in 'Keitt' mango, Shaban et al. (2021) found no sunburn damage when covering trees with a white shading net (40% shading), associated with an irrigation level of 70% (Tab. 1).
Araújo and Britto (2021) evaluated the intensity of sunburn damage on harvested mango fruits treated with bentonite clay and kaolin exposed to incandescent irradiation. According to the authors, bentonite clay provided better protection to fruit sunburn than kaolin.
Pineapple
The reduction of direct radiation is a common practice in pineapple cultivated in the tropics to protect fruits against sunburn, characterized by translucent pulp appearance (Restrepo-Díaz and Sánchez-Reinoso, 2020), which can generate losses up to 70% of fruit production (Lopes et al., 2014) (Fig. 3).
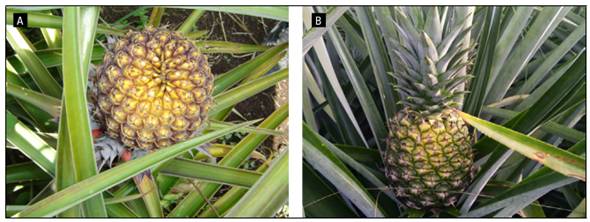
Photos: G. Fischer (A) and M.T. Pulido (B).
Figure 3. Sunburn on pineapple fruit (near Pereira, Colombia, 1,400 m a.s.l.) (A) and on cv. Gold in the Ariari region (Meta, Colombia), 350 m a.s.l. (B).
Zhaoet al. (2020) reported in Golden (MD-2) pineapple best fruit quality with little incidence of sunburn (Tab. 1) by covering the plants (at 0 cm) with blue shading net (70% light transmittance) 30 d after flower fading. In 'Perola' pineapple, Teixeira et al. (2022) observed that the application of CaCO3 nanoparticles on fruits, with increasing concentrations from 12.5 to 200 g ha-1 (Tab. 1) decreased sunburn, yellowing index and heating, and improved fruit quality (higher firmness, TSS/ATT ratio and ascorbic acid content) and postharvest life. These authors assumed that the increased ascorbic acid content of the fruit, because of its antioxidant potential, reduced the photooxidative damage.
In Perola pineapple, Lopes et al. (2014) studied the protection of fruits with different bags (made with newspaper, brown paper, and TNT white nº 40) and by spraying 10% lime solution, associated with five irrigation regimes (between 30 and 150%, based on Class A Evaporation Pan) on fruit quality. The authors found the lowest percentage of sunburn (23%) in fruits covered with the TNT bag, which also guaranteed the highest percentages of TSS when applying irrigation of 77% according to Pan.
Pitaya
These cacti fruit and other Hylocereus species are native to the tropical forests of the Americas (Nobel and de la Barrera, 2002), so they suffer from high solar radiation and temperatures in commercial plantations (Oliveira et al., 2021). Besides the fruit, sunburn can also affect other organs of pitaya, such as the cladodes (Fig. 4), which justify the protection of first plantations of red pitaya with shading (Oliveira et al., 2021).
In red pitaya, protection with shading nets (providing 25 to 75% shade) increased chlorophyll content and green color and decreased sunburn of the cladodes, while black shading net (50% shade) increased fresh weight and reduced cracking of the fruit (Tab. 1) (Chang et al., 2016). Oliveira et al. (2021) covered red pitaya (Hylocereus costaricencis) plants with black nets providing between 35 and 80% shade. The authors reported better physiological performance in plants covered with nets, especially with the net providing 35% shade, with an increase in yield (70%) and better fruit quality (increase of fresh weight, firmness and red pulp coloration by betalain pigments) compared to plants in full sunlight, in the second year of the study. In addition, the cladodes of plants covered with nets had higher total chlorophyll content and no symptoms of sunburn or photoinhibition.
Other tropical and subtropical fruits
Sunburn has been reported in many other fruit trees but without detailed studies. For passionfruit species cultivated in Colombia, sweet granadilla (Passiflora ligularis) is highly susceptible to fruit sunburn by lacking leaves to protect the fruit from excessive solar radiation (Fig. 5), a similar situation was observed for purple passionfruit (P. edulis f. edulis) (Fischer and Miranda, 2021). For yellow passionfruit (P. edulis f. flavicarpa), Cleves et al. (2012) recommended 5 h of daily sunshine, which increases TSS and ascorbic acid concentrations in the fruit, but this increases the risk of fruit sunburn. To prevent fruit sunburn, growers should avoid severe pruning, especially near harvest, removing fruit with pests and diseases (Fischer et al., 2009). In banana passionfruit (P. mollissima f. tripartita) the thick epidermis protects the fruit from UV radiation at tropical altitude (Fischer and Miranda, 2021), which increases its resistance to diseases and sunburn.
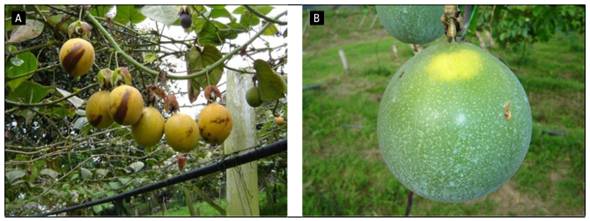
Photos: G. Fischer.
Figure 5 The lack of protective leaves causes sunburn on sweet granadilla (near Silvania, Cundinamarca, Colombia, at 1,400 m a.s.l.) (A) and on yellow passion fruit (La Unión, Valle, Colombia, at 1,000 m a.s.l.) (B).
In solanaceous fruit trees, there is low risk of sunburn from high UV radiation in the tropical highlands. Lulo (or naranjilla; Solanum quitoense) has a dense pubescence that protects the entire plant and the fruit from high radiation, but in extreme conditions sunburn on fruits was observed, while the cape gooseberry (Physalis peruviana) fruit is completely protected by the calyx, and in tree tomato (Solanum betaceum), fruit has high concentration of anthocyanins that protect it from sunburn (Fischer and Melgarejo, 2020; Fischer et al., 2021b). There is an increase in the number of stomata on the epidermis of the fruit at tropical altitude to compensate for the reduced partial pressure of oxygen and CO2 (Körner, 2007; Fischer et al., 2022b). This was reported by Fischer and Melgarejo (2020) for the cape gooseberry, that might decrease fruit surface temperature as a result of increased fruit transpiration.
For myrtaceae species, feijoa fruit (Acca sellowiana) has high content of polyphenols that protect it from sunburn (Saric and Sivamani, 2016), and extracts from this fruit have a medicinal use for sun skin protection (Ebrahimzadeh et al., 2014); however, fruit not covered by leaves also suffer solar injuries (Fig. 6). In the case of guava (Psidium guajava), varieties susceptible to fruit flies in Colombia (e.g. ‘Manzana’, ‘Calima’, ‘Indian Pink’ and ‘Pear’) have the fruit bagged with blue PE bags (Fig. 7), which also protects from sunburn (Fischer et al., 2012).
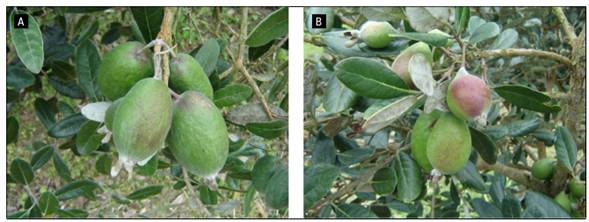
Photos: G. Fischer.
Figure 6. Sunburn in feijoa fruits not protected by leaves (Duitama, Boyaca, Colombia, 2,450 m a.s.l.) at the beginning of the damage (A) and with more advanced damage (B).
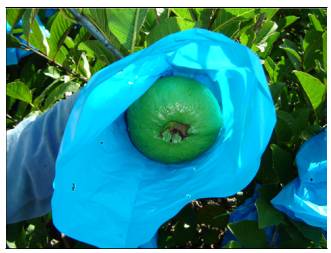
Photo: G. Fischer.
Figure 7. Bagging of the ´Manzana´ guava fruit with blue PE bags as protection against the fruit fly and sunburn (La Unión, Valle, Colombia, 1,000 m a.s.l.).
Sunburn damage also occurs in umbrophilous plants, such as borojó (Borojoa patinoi), when fully exposed to solar radiation. According to the second author's observations, borojó fruits cultivated in the foothills of Meta (Colombia) are not affected by sunburn when they grow and ripen during the rainy season (April to November). However, during the dry season (December to March), when plants suffer from water deficit and loss of foliage and with temperature increase and atmospheric humidity decrease, fruits suffer severe sunburn (Fig. 8). The damage occurs at the angle of the sun's fall in the afternoon and in the westerly direction when the sun hits the fruit perpendicularly.
CONCLUSIONS
One of the effects of climate change and variability (with excessive radiation and increased temperature) is fruit sunburn. This is aggravated by low relative humidity conditions, in fruit left unprotected by tree foliage. Sunburn is of a great concern on plantations at higher altitudes, exposed to high UV radiation, or when a dry and sunny climate follows a humid and cold period. Sunburn highly affects fruit marketability and, thus, productivity of the orchard.
Fruit protection against abiotic stresses occurs through the production of enzymatic and non-enzymatic antioxidants. Mitigation strategies of fruit growers to prevent fruit sunburn include selection of tolerant varieties, pruning and management of plants, leaving enough leaves above fruits, efficient irrigation or tree shading with intercropping. Other technical strategies to mitigate the effects of sunburn include orchard protective measures (shading nets, fruit bagging, evaporative cooling), suppressors (kaolin clay particle films and calcium carbonate) and chemical protection (tocopherol, abscisic acid, ascorbic acid, anti-transpirants).
This paper covers the state of the research on sunburn disorder for some tropical and subtropical fruit trees, such as avocado, banana, citrus, mango, pineapple and pitaya, with observations for other fruits growing at tropical altitudes. Continued research is recommended with different species and varieties of fruit trees under conditions of high radiation and temperature to find tolerance mechanisms that can be used with environmentally friendly protective materials. For plant breeding, studies are required on molecular mechanisms that direct the acclimatization of plants to a combination of these two types of stress, excessive radiation and high temperature.