INTRODUCTION
Lettuce is a widely consumed vegetable worldwide, with great commercial availability because of its short growth time and nutraceutical quality given its content of ascorbic acid, carotenoids, flavonoids, and tocopherols, favoring human health (Hong et al., 2022). In 2021, approximately 27.7 million t of lettuce were produced worldwide, with China having the highest production (51.8%), followed by the United States with 15.9% and India with 4.1% (FAO, 2021). Production in Colombia was 97,240 t in 2016, with a yield of 21.95 t ha-1, where production is concentrated in the departments of Cundinamarca, Antioquia, and Nariño given their large planting areas (Agronet, 2023).
It is estimated that the global population will increase by between two and three billion by 2050 and that half of the population will be fed with crops grown with nitrogen fertilizer (Zhang et al., 2015). Likewise, according to the UNEP (2019), excess nitrogen fertilization has increased N levels both in the soil and water, altering life. N contributes greatly to climate change and constitutes a threat to human health, leading to the need for careful management of nitrogen and fertilizer elements, such as applying adequate magnesium to satisfy crop requirements and nourish a growing population, while minimizing environmental effects.
Crop yield and quality are directly related to the supply of mineral nutrients during the growth stages, with nitrogen (N) and magnesium (Mg) being essential for lettuce cultivation (Mahlangu et al., 2016). N plays an important role in chlorophyll content and photosynthetic capacity; therefore, a N deficiency reduces productivity (Gong et al., 2020; Hong et al., 2022) by decreasing the development of the leaf area, as well as photosynthetic assimilation, which decreases the source of carbon skeletons, important characteristics in the commercial production of leafy vegetables such as lettuce (Mahlangu et al., 2016). According to Coulombier et al. (2020), the adequate availability of N increases the contents of B1 vitamins and carotenes such as lutein, beta-carotenes, neoxanthin, and zeaxanthins, in addition to regulating the biosynthesis of secondary metabolites such as phenols.
Likewise, Mg is a constituent of chlorophyll; therefore, it is important to the processes of photosynthesis, enzymatic activation, production, and transport of photoassimilates. In addition, it serves as a link between substrates and, in combination with ATP, stabilizes ribosomal particles, acting as an indicator of enzymes involved in protein synthesis (Ishfaq et al., 2022). Hence, the growth and development of plants can be seriously inhibited by its deficiency, which generates detrimental effects on the productivity and quality of crops (Chen et al., 2018).
Therefore, the objective of this work was to evaluate the growth and production of lettuce cv. Vera crops subjected to different doses of nitrogen and magnesium to provide producers with optimal fertilization management with these nutrients.
MATERIALS AND METHODS
Location and plant material
This study was carried out under a plastic roof in the San Francisco zone, municipality of Mosquera-Cundinamarca, at 4°41'27” N and 74°12 09” W, with an altitude of 2,542 m. Laboratory analyses were carried out in the Plant Physiology Laboratory of the National University of Colombia. The plastic greenhouse had a chapel-type structure, with an area of 160 m2 and a maximum height of 6 m, and 80% white polyshade was used to control light intensity. Crispy lettuce Vera variety seedlings from a Rioplant certified propagator were sown in three 1.2 m wide by 12 m long beds, with a planting distance of 0.3 m between plants and 0.3 m between rows.
The physical and chemical properties of the soil are in Tab. 1. Fertilization was carried out according to the nutritional requirements of the crop in an edaphic form divided into two parts. The first fertilizer application was made seven days after transplanting (dat), and the second was done at 20 dat, incorporated into the crown. The nitrogen and magnesium were applied according to Tab. 1. In addition, 70 kg ha-1 of P2O5, 200 kg ha-1 of K2O, 51 kg ha-1 of CaO, and 10 kg ha-1 of S were added, using as source potassium sulfate, potassium chloride, dicalcium phosphate, triple superphosphate, magnesium nitrate and urea. Agronomic management for pests, diseases and weeds was carried out opportunely according to the monitoring.
Table 1. Physical and chemical analysis of the soil from the municipality of Mosquera, Cundinamarca.
pH | EC (dS m-1) | OC | N | Ca | K | Mg | Na | EAl | ECEC | |
---|---|---|---|---|---|---|---|---|---|---|
% | meq 100 g -1 | |||||||||
5.50 | - | 3.33 | 0.29 | 19.60 | 0.18 | 3.46 | 3.29 | 0.00 | 26.50 | |
Particle size | Clay | Silt | Sand | P | S | Cu | Fe | Mn | Zn | B |
% | mg kg-1 | |||||||||
Clay loam | 35 | 43 | 22 | 55.5 | 149 | 0.78 | 76.3 | 19.7 | 31.8 | 0.41 |
EC: electric conductivity; OC: organic carbon; EAl: exchangeable aluminum; ECEC: effective cation exchange capacity.
Experiment design
A randomized complete block design was developed with ten treatments: a control plus nine treatments with a central compound (Tab. 2) according to Martínez (1988). Each block was equivalent to a replicate, for a total of 30 experiment units (EU), and each EU contained 16 plants, for a total of 480 plants.
Table 2. Treatment combination codes for two factors in a central composite design.
Treatment structure | Number of treatments | X1 | X2 | Doses of N (kg ha-1) | Doses of Mg (kg ha-1) |
---|---|---|---|---|---|
Control | 1 | - | - | 0 | 0 |
Basic factorial | 2 | -1 | -1 | 50 | 6 |
3 | -1 | +1 | 50 | 18 | |
4 | +1 | -1 | 150 | 6 | |
5 | +1 | +1 | 150 | 18 | |
Axial point | 6 | -α | 0 | 0 | 12 |
7 | +α | 0 | 200 | 12 | |
8 | 0 | -α | 100 | 0 | |
9 | 0 | +α | 100 | 24 | |
Central point | 10 | 0 | 0 | 100 | 12 |
Response variables
Samplings were carried out every 8 d for the relative chlorophyll content and every 15 d for the other variables, taking three random plants per treatment and per block, for a total of 360 plants evaluated in four samplings at 21, 35, 49, and 60 dat. The leaf area (LA) was measured by taking photos of the fully extended leaves, which were analyzed according to the methodology recommended by Schneider et al. (2012) using ImageJ version 1.45. The relative chlorophyll content was determined with a SPAD-502 Plus (Konica Minolta, Inc., Tokyo, Japan). The fresh and dry mass were measured with an Adam PGW-2502e electronic balance (Adam Equipment Co. Ltd., Milton Keynes, UK) with a precision of 0.01 g and a Memmert drying oven, model UNB500 (Memmert GmbH + Co. KG, Schwabach, Germany), at a temperature of 75°C for 72 h.
The following growth indices were calculated: leaf area ratio (LAR), relative growth rate (RGR), net assimilation rate (NAR), agronomic efficiency, and partial productivity factor, according to Tab. 3.
Tabla 3. Growth and efficiency rates.
Index | Equation |
---|---|
Leaf area ratio(LAR) |
|
Relative growth rate (RGR) |
|
Net assimilation rate (NAR) |
|
Agronomic efficiency (AE) |
|
Partial factor productivity (PFP) |
|
W: dry mass; T: time; LA = leaf area; ln: natural logarithm; Y: yield with applied fertilizer; Y0: yield without fertilizer input; F: amount of fertilizer applied; ΔY: incremental yield that results from de fertilizer application.
Data analysis
The data were subjected to a normality test, analysis of variance (Anova), and Tukey test (P≤0.05) to establish differences between treatments and classify them. For the performance variable, a response surface analysis was performed to establish the optimal combination of N and Mg that generated the maximum yield values. This model was fitted to a quadratic function for the factorial design with SAS version 9.4 (SAS Institute Inc., Cary, NC).
RESULTS AND DISCUSSION
Leaf area (LA) and Leaf area ratio (LAR)
There were only significant differences for LA between treatments at 21 and 35 dat (Fig. 1A). However, a significant and progressive increase was observed over time, reaching a maximum of 1,753 cm2 with 150 kg ha-1 N + 6 kg ha-1 Mg at 60 dat, and the treatments in general had a significant average value of 1,533 cm2, which is higher than the 902.3 cm2 reported by Cho et al. (2018) in 'Cheongchima' lettuce subjected to a light intensity of 200 μmol m-2 s-1. It should be noted that one of the main functions of leaves is the interception of solar radiation to carry out the photosynthesis process, where a greater LA allows a greater absorption of radiation and thus achieve maximum physiological development (Frąszczak and Kula-Maximenko, 2021). These authors found that lettuce with different colors and thicknesses intercepted different spectra of light.
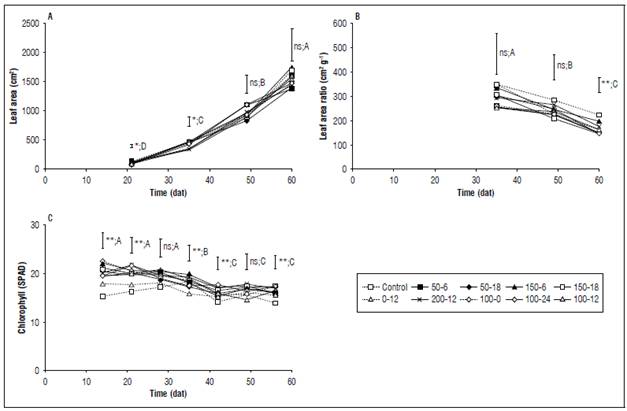
Figure 1. A) Leaf area, B) leaf area ratio, and C) chlorophyll (SPAD) of the 'Vera' lettuce crop subjected to different doses of nitrogen and magnesium. ns: not significant, * and ** indicate significant effect according to the Anova (P≤0.05 and P≤0.01, respectively) between the treatments before the semicolon, and different uppercase letters after the semicolon indicate significant differences between measurements over time according to Tukey (P≤0.05). Vertical bars indicate the minimum significant difference between treatments for the Tukey test.
On the other hand, the LAR decreased over time (Fig. 1B), going from a maximum value at 35 dat of 296.07 cm2 g-1 to 165 cm2 g-1 at 60 dat, at which time the control treatment presented the highest LAR value with 222 cm2 g-1, which indicated greater efficiency in the generation of LA with a lower dry mass requirement. This response can be related to the production of photoassimilates, required in greater quantities during the early stages of development in photosynthetically active parts, which increases energy expenditure; consequently, a lower mass was obtained, which is consistent with Simkin et al. (2020) who found that crop yield is determined by the cumulative rate of photosynthesis during the growing season.
Relative chlorophyll content
There were significant differences between treatments and between the different measurement times, however, the interaction did not have significant differences (Fig. 1C). The SPAD values varied over time and decreased from 19.7 at 14 dat to 16.26 at 56 dat, which were lower than the values of 26 to 35 obtained by Liu et al. (2014) and from the 24.1 to 30.1 values observed by Cho et al. (2018) by varying the light intensity from 100 to 200 μmol m-2 s-1, respectively, and higher than those reported by Frąszczak and Kula-Maximenko (2021), with oscillated between 2 and 12. Likewise, these authors stated that the application of blue light in lettuce reduced the SPAD content.
Higher chlorophyll contents were found in SPAD units at 14 dat, while lower values occurred at 49 and 60 dat, which coincided with the lowest applications of magnesium fertilization (Figure 1C). Mg is the central atom of the chlorophyll molecule, is fundamental in photosynthesis, is also involved in protein synthesis and has an important role in energy transfer (Peng et al., 2019). Therefore, the decrease in the concentration of chlorophylls measured in the SPAD units could be due to the fact that the contents of N and Mg decreased with the age of the plants because, during the growth and development of crops, the size and number of leaves increases and, therefore, the requirements of these elements are increased by photosynthetic activity, where N applications increase the photosynthetic rate (Leghari et al., 2016).
Net assimilation rate (NAR)
The NAR showed significant differences between treatments only at 50 dat, where the application of 150 kg ha-1 N + 18 kg ha-1 of Mg showed higher values (Fig. 2A), 77.4% higher than the control treatment, which had the lowest NAR. Despite the fact that the measurements and the interaction did not present significant differences over time for the NAR, it increased until 49 dat, subsequently decreasing slightly until the end of the cycle at 60 dat. This reduction NAR is related to the behavior of LA because leaves are more exposed to radiation and are more efficient in the uptake and assimilation of CO2 during the early stages of development; therefore, an adequate supply of nitrogen fertilization in the first stages will be reflected in a greater LA, greater photosynthetic capacity and greater chlorophyll content (Mahlangu et al., 2016). Likewise, as plants develop, external leaves increase shading on internal leaves, which translates into a reduction in the capture of photosynthetically active radiation and affects photosynthetic rates according to Chapepa et al. (2020), who reported that attenuation in light capture is affected by canopy architecture.
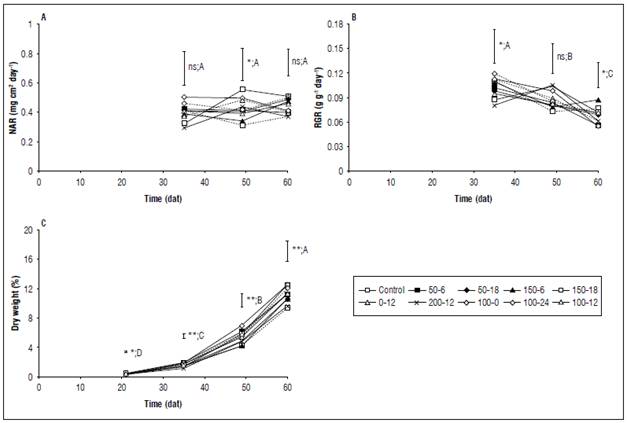
Figure 2. A) Net assimilation rate (NAR), B) Relative growth rate (RGR) and C) Dry weight of the crop of lettuce 'Vera' subjected to different doses of nutrition with Nitrogen and Magnesium. ns: not significant, * and ** indicate significant effect according to the Anova (P≤0.05 and P≤0.01, respectively) between treatments before the semicolon, and different uppercase letters after the semicolon indicate differences significant between measurements over time according to Tukey (P≤0.05). Vertical bars indicate the minimum significant difference between treatments of the Tukey test.
The constant increase or increase following a decrease in the NAR in some treatments was explained by the fact that it is possible that the concentration of assimilates, and the generation of dry biomass were higher because of a higher production and better distribution of leaves and, as a consequence, because a reduction of photosynthetic activity was not seen (Frąszczak and Kula-Maximenko, 2021). It is also probable that having a greater availability of nutrients resulted in optimal distribution of photoassimilates in the different plant organs (Leghari et al., 2016).
Relative growth rate (RGR)
There were significant differences between interaction at 35 and 60 dat for RGR (Fig. 2B). At 35 dat, the control treatment showed the best results, while at 60 dat, the application of 150 kg ha-1 of N + 6 kg ha-1 of Mg reached the highest RGR data. In the same way, the measurements had significant differences, and the RGR decreased during the entire crop cycle; at 35 dat, it was 51.26% higher than at 60 dat, going from 0.102 to 0.067 g g-1 d-1. These results are similar to those obtained by Martínez and Garcés (2010) in ‘Romana’ variety lettuce, who, at 40 dat, found values of 0.117 g g-1 d-1 in plants subjected to 180 mg L-1 of potassium. The RGR is important because it refers to the growth speed of the plant in relation to its current biomass; likewise, it determines the time it takes for the plant to reach harvest. Generally, low RGR rates are indicators of the presence of some type of stress (El-Nakhel et al., 2019).
Dry biomass
There were significant differences between interaction and between the different measurement times for the accumulation of dry biomass (Fig. 2C). The highest values of dry mass were obtained in the treatments with applications of 100 kg ha-1 N + 24 kg ha-1 Mg and 150 kg ha-1 N + 18 kg ha-1 Mg with 12.52 and 12.50%. In this regard, Liu et al. (2014) stated that the application of 200 kg ha-1 N (0.39 g/plant) in lettuce saw a higher value than the dose of 400 kg ha-1 N (0.12 to 0.31 g/plant) and the control (0.14 g/plant) in terms of accumulated dry biomass at 50 dat. Similarly, Cho et al. (2018) reported that the dry mass at 20 dat reached values of 3.03 g/plant under light intensities of 200 μmol m-2 s-1.
In this regard, it is estimated that a plant requires 1.5 to 3.5 g kg-1 of dry mass of Mg, which is used in numerous physiological and biochemical processes (Chen et al., 2018), of which, between 15 and 35% of the plant's Mg binds to chloroplasts, mainly as a component of chlorophyll where it is key in the energy transfer process (Chen et al., 2018). Furthermore, a Mg deficiency decreases the photosynthetic rate and blocks carbohydrate allocation to sink organs, which inhibits growth and ultimately leads to low productivity in many plant species (Yang et al., 2012).
Yield
There were significant differences between interactions for crop yield. The highest yield was obtained with the application of 150 kg ha-1 of N + 18 kg ha-1 of Mg with 22.59 t ha-1, which was 51.15% higher than the yield of the control, which, in turn, was the treatment with the lowest value (Fig. 3A). It should be noted that an adequate supply of N positively increases its absorption and, consequently, the production of leaves and their size, as reported by Hong et al. (2022) who found increases in production between 13% and 22% when they increased the application of N to 315 kg ha-1. Likewise, cell division and expansion increase the growth rate of leaves; therefore, a nutritional deficit directly affects the production of new leaves and leaf growth (Martínez and Garcés, 2010). Regarding the contribution of Mg to production, Peng et al. (2019) reported that this element is relatively mobile since it translocates from old to young tissues in plants under limited conditions, being stored in greater quantity in the latter.
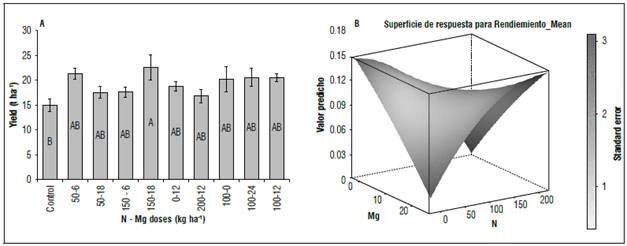
Figure 3. A) Yield and B) response surface plot of yield of the crop of 'Vera' lettuce subjected to different doses of nutrition with nitrogen and magnesium. Different uppercase letters indicate differences significant between treatments according to Tukey test (P≤0.05). Vertical bars indicate the minimum significant difference between treatments of the Tukey test.
Multiple linear regression was plotted as a response surface (Fig. 3B), which had a degree of fit of 0.942 with a coefficient of variation of 3.69%. The adjusted regression model was the quadratic one, being significant for the treatments (P=0.0479), the interaction (P=0.0145), and for each of the independent factors, N and Mg (P =0 0.0322 and P=0.0492, respectively). The optimum application level was estimated with the regression equation, which showed that fertilization should be carried out with 93.8 kg ha-1 N and 12.4 kg ha-1 Mg to obtain the maximum yield (21 t ha-1). These N doses were relatively low because Liu et al. (2014) applied 315 kg ha-1 N and obtained a production of 15 t ha-1, which was lower because the present trial also used Mg fertilization, which may have increased production.
Agronomic efficiency (AE) and Partial Factor Productivity (PFP)
The highest AE for N occurred with the application of 50 kg ha-1 of N + 6 kg ha-1 of Mg since it managed to increase yield by 126 kg when compared to the control treatment (Tab. 4). In the case of Mg, the highest AE was obtained with the dose of 50 kg ha-1 of N + 6 kg ha-1 of Mg with a 1051 kg increase in yield compared to the control, per kg of Mg applied, which coincided with the second highest yield of 21.2 t ha-1, which indicated that, by only applying 6 kg of Mg, high production efficiency was already achieved. These values were higher than those of Guelfi-Silva et al. (2013), who reported AE values that ranged between 12 and 19 kg kg-1 of potassium with 200 kg ha-1 in a lettuce crop.
Table 4. Yield, dry mass, agronomic efficiency (AE) and partial factor productivity (PFP) of the crop of 'Vera' variety of crisp lettuce.
N | Mg | Yield | Dry mass | Relative yield | AE (kg increment/kg element) | PFP (kg FW/kg element) | ||
---|---|---|---|---|---|---|---|---|
(kg ha-1) | (%) | N | Mg | N | Mg | |||
0 | 0 | 14,944.4 | 66 | 6.42 | - | - | - | - |
50 | 6 | 21,255.6 | 94 | 5.58 | 126.2 | 1051.9 | 425.1 | 3,542.6 |
50 | 18 | 18,855.6 | 83 | 5.90 | 78.2 | 217.3 | 377.1 | 1,047.5 |
150 | 6 | 17,577.8 | 78 | 6.07 | 17.6 | 438.9 | 117.2 | 2,929.6 |
150 | 18 | 22,588.9 | 100 | 5.95 | 51.0 | 424.7 | 150.6 | 1,254.9 |
0 | 12 | 18,711.1 | 83 | 6.10 | - | 313.9 | - | 1,559.3 |
200 | 12 | 16,755.6 | 74 | 5.81 | 9.1 | 150.9 | 83.8 | 1,396.3 |
100 | 0 | 20,166.7 | 89 | 6.37 | 52.2 | - | 201.7 | - |
100 | 24 | 20,577.8 | 91 | 6.33 | 56.3 | 234.7 | 205.8 | 857.4 |
100 | 12 | 20,500.0 | 91 | 5.82 | 55.6 | 463.0 | 205.0 | 1,708.3 |
FW: fresh weight.
On the other hand, when analyzing the PFP for the two elements, the application of 50 kg ha-1 of N and 6 kg ha-1 of Mg obtained higher values with a PFP of 425.1 and 3,542.6 kg kg-1 for N and Mg, respectively (Tab. 4). When comparing this treatment with the one that registered the highest production (150 kg ha-1 of N and 18 kg ha-1 of Mg), it can be seen that the PFP was 182% higher, which implied that adding more fertilization did not improve performance linearly, in accordance with the law of diminishing returns or Mitscherlich's law (Hasnain et al., 2020). Similarly, the values were similar to the PFP reported for lettuce, which ranged from 78 to 252 kg kg-1, 292 to 885 kg kg-1 and 82 to 190 kg kg-1, for N, P, and K, respectively (Sangare et al., 2012).
CONCLUSION
The leaf area ratio, SPAD chlorophyll index, and relative growth rate decreased over time during the crop cycle. The application of 150 kg ha-1 of N + 18 kg ha-1 of Mg generated the highest production and NAR values. According to the response surface graph, fertilization with 93.8 kg ha-1 of N and 12.4 kg ha-1 of Mg should be carried out to obtain maximum yield in 'Vera' lettuce. The treatments with lower applications of both nitrogenous and magnesium fertilization showed higher values of agronomic efficiency and partial productivity factor.