INTRODUCTION
The avocado (Persea americana Mill.) belongs to the Lauraceae family and is distributed between 0 and 2,200 m a.s.l. This species is cultivated in more than 50 countries worldwide, in tropical and subtropical regions (Montes-Hernández et al., 2017; Hurtado-Fernández et al., 2018). Colombia is in the second position of the world ranking of Hass avocado producers, with 979,618 tons produced in 2021, only behind Mexico, the largest producer, with 2.44 million tons (FAO, 2022).
As an allogamous and highly heterozygous plant, propagation from seeds originates in plants that present high variability in elite characters for orchard characteristics. For this reason, grafting as a method of propagation and production of high-quality grafted plants with high production capacity and tolerance to biotic and abiotic constraints is today the most used for the establishment of avocado cultivation in producing countries (Tripathi and Karunakaran, 2019). In this context, the Guatemalan, Mexican, and Antillean or West Indian races have become the primary seed source for rootstock production, mainly in countries such as Chile (Castro et al., 2008), Brazil, and Mexico, where plants with high vigor and hardiness originate (Alberti et al., 2017).
In Colombia, seed from native trees that are widely distributed throughout the territory is used and characterized by great diversity due to natural hybridizations between races of P. americana, allowing these genotypes to adapt to a great variety of environmental conditions (Ramírez-Gil, 2018). The selection of a suitable avocado cultivar as a source of rootstock seeds is considered of great importance since the success or failure after the establishment of the plantation depends on the attributes of the material used as a rootstock in the production of grafted plants (Barrientos-Priego et al., 2007). The avocado horticulturists do not know the origin of the seed used as a rootstock due to the high heterosis of P. americana as a cross-pollinated species, generating genetically uneven rootstocks with significant morphological and physiological variability limits attributes in establishing commercial orchards (Petry et al., 2012). Thus, although a commercial scion with outstanding characteristics is used when grafted on rootstocks obtained from seeds of unknown origin, the grafted plants differ in size, yield, quality (caliber), physiological performance and tolerance to different abiotic factors, and resistance to biotic ones (Petry et al., 2012).
Most commercial avocado Hass plantations in Colombia areas are currently based on open-pollinated (OP) half-sib interracial seedling rootstocks derived from selected creole “elite trees”. So avocado nursery trees are traditionally based on grafting the commercial cv. Hass onto untested creole seedling rootstocks obtained from non-grafted, highly heterozygous, and vigorous avocado native trees, resulting in sizeable inter-tree variability within the commercial orchards (Bernal et al., 2014; Cañas-Gutiérrez et al., 2015). For the above, the physiological and morphological characterization of creole rootstocks to understand the variation and identify best-performing individuals is a valuable resource that can be used to minimize yield variations and to achieve homogeneity of avocado orchards. This study aimed at morphological and physiological characterization of creole avocados previously identified as outstanding cultivars to identify elite rootstocks.
MATERIALS AND METHODS
Location: The work was developed in the CARTAMA avocado nursery in the municipality of Supía (Caldas) at 5°26'39'' N; 75°38'56'' W, and 1,185 m a.s.l. The average temperature is between 22 and 24°C, a minimum of 17.4°C and a maximum of 28.1°C, with average annual rainfall (1,117 mm), and maximum relative humidity of 100%, and a minimum of 53.5%.
Genetic resource: The genetic materials evaluated with potential for rootstocks came from seed introductions made by AVOFRUIT S.A.S. from the municipalities of Mutata, Santa Barbara, Sonson, Abejorral, Urrao, Nariño in Antioquia, and Norcasia, Samana, Corregimiento de Berlin, La Merced, Salamina and Aguadas in Caldas. The edaphoclimatic characteristics are shown in table 1.
Experimental design: A completely randomized experimental design was used with 17 treatments (identified with the code ID-01 to ID-17) and 20 replications. Each treatment consisted of one avocado creole tree accession as a source of rootstock seeds from different avocado-producing regions in Colombia (Tab. 1). The avocado accessions were determined according to quality references provided by avocado farmers. The best agronomic expressions supported the Creole accessions regarding rootstock height, longevity, vigor, yield, little alternation, earliness, behavior against pests and diseases, and tolerance to water excesses and deficits.
Table 1. Environmental characteristics of the localities where the creole avocado rootstocks were collected.
Department | Municipality | Annual rainfall (mm) | Tmax1 (°C) | Tmin2 (°C) | RH3 (%) | ETo4 (mm) | Life zone | Soil texture |
---|---|---|---|---|---|---|---|---|
Antioquia | Urrao | 2,360 | 24.8 | 14.4 | 93 | 1,144 | vwf-T | Silty loam |
Antioquia | Sonsón | 2,052 | 28.6 | 8.7 | 60 | 1,275 | vwf-PM | Medium and fine |
Antioquia | Nariño | 1,216 | 25.0 | 16.0 | 83 | 1,170 | vwf-PM | Medium and fine |
Antioquia | Betulia | 1,706 | 26.6 | 10.1 | 85 | 1,050 | wf-PM - vwf-PM | Fine and moderately fine |
Antioquia | Santa Barbara | 2,383,9 | 25.8 | 13.2 | 94 | 882 | wf-PM - vwf-PM | Fine and moderately fine |
Antioquia | Mutatá | 5,474.4 | 29.3 | 10.7 | 95 | 1,807 | vwf-T | Fine and medium |
Antioquia | Abejorral | 2,235.4 | 25.7 | 11.2 | 86 | 786 | wf-T | Medium and fine |
Caldas | Samaná | 3,001-7,000 | 26.5 | 17.0 | 80 | 536 | wf-PM - wf-LM | Fines and medium (clay-loam) |
Caldas | Berlín | 2,397 | 27.0 | 20.0 | 69 | 538 | wf-LM | Clay loam (predominant) |
Caldas | La Merced | 1,730 | 24.0 | 19.0 | 68 | 538 | vwf-PM | Clay loam and sandy loam |
Caldas | Salamina | 2,397 | 20.0 | 15.0 | 67 | 565 | vwf-PM | Clay loam and sandy loam |
Caldas | Pacora | 1,500-3,000 | 21.0 | 14.0 | 67 | 538 | vwf-PM | Clay loam and sandy loam |
Caldas | Norcasia | 4,001-5,000 | 30.0 | 22.0 | 66 | 538.46 | vwf-T | Clay loam (predominant) |
Caldas | Aránzazu | 1,501-2,500 | 21.0 | 13.0 | 72 | 514 | vwf-PM | Clay loam (predominant) |
1Tmax (maximum temperature), 2Tmin (minimun temeperature), 3RH (Relative humidity), 4Eto (Evapotranspiration). Tropical very wet forest (vwf-T), Premontane very wet forest (vwf-PM), Premontane wet forest (wf-PM), Tropical wet forest (wf-T),Lower Montane wet forest (wf-LM).
The seeds were extracted from mature and healthy fruits, which were disinfected before the pre-germination process. Later the seeds were transplanted into black bags (15 d later, in April and May) of caliber 3.7 with, 50 cm high and 15 cm wide, and with perforations and micro-perforations. The substrate was a mixture of 70% soil, 20% sand, and 10% coffee hull, disinfected with 50 g of basamid (1.2 g per bag). Based on the properties and characteristics of the substrate, the nutrition of the rootstocks was carried out through fertigation with the application of N (1.31 mg), P2O5 (3.65 mg), K2O (1.3 mg), MgO (0.04 mg), CaO (0.27 mg), S (0.52 mg), B (0.002 mg), Cu (0.0035 mg), Fe (0, 0004 mg), Mn (0.000713 mg), Mo (0.00008 mg), and Zn (0.0003 mg) per plant.
Gas exchanges: The gas-exchange measurement was done over the seedling (rootstocks) fifth fully expanded young leaves, with the portable infrared gas analyzer LCi - ADC (Bioscience, UK), provided by an external halogen lamp ADC (Bioscience, UK) under a saturating photosynthetic photon flux density (PPFD) of 1,100 μmol m-2 s-1. The gas-exchange variables determined were the net photosynthesis - A (μmol CO2 m-2 s-1), stomatal conductance - gs (mol H2O m-2 s-1), and transpiration rate - E (mmol H2O m-2 s-1). Water-use efficiency (WUE) was estimated as the ratio between A and E (μmol CO2/mmol H2O).
Dry matter: the biomass of three plants per rootstock was harvested, from which the dry weight per organ (leaves, stems, roots, and seeds) was determined; for this, each organ was individually placed in drying ovens (Memmert type UL 80) at 70°C, until reaching constant weight. The final weight was considered as the phytomass in each of the structures, and the sum of them was the total dry weight of the plant. LAI was estimated by direct and destructive methods, according to Fang et al. (2019). LAI was directly obtained by harvesting rootstock leaves through destructive sampling, and the leaf area (LA) was measured using a portable Li-Cor (Li-3000) leaf area meter. Specific leaf area (SLA - leaf area per unit of dry leaf mass) was determined according to Baret et al. (2010), as the relation of leaf area/leaf dry matter.
Vegetative growth: The measurements of growth variables were carried out in non-grafted rootstock seedlings at 90 days after transplanting the germinated avocado seed to a plastic bag when the rootstock was considered suitable for grafting. The variables evaluated were stem diameter (cm), rootstock height (cm), leaf number, leaf area (cm2), leaf area index (LAI), and specific foliar area (SFA).
Statistical analysis: Two-way analysis of variance (ANOVA) was performed to reveal differences within each treatment (rootstock) after validation of the assumptions of normality and homoscedasticity (Shapiro-Wilk and Bartlett tests). For the mean comparison, an HSD-Tukey test was performed (level of 5%) using the R project "agricolae" package (de Mendiburu, 2021).
Additionally, a principal component analysis was used to determine the variables that have the most weight on the characteristics evaluated and to determine those valid for the discrimination of genotypes. Using the R program, determining the characters responsible for the greatest variability in the varieties was obtained through the multivariate cluster analysis, considering the Euclidean distance between the clusters. This analysis aims to identify the genotypes with the highest and least variation within each phenotypic group.
RESULTS AND DISCUSSION
Avocado rootstock breeding programs have been focused on performing a selection for resistance to soil-borne pathogens, especially to respond to Phytophthora cinnamomi infection (Fick et al., 2022). Nevertheless, yield, physiological, and morphological adaptive traits are considered as the second and third steps within avocado rootstock breeding (Cañas-Gutiérrez et al., 2022). Thus, avocado nurseries often rely on clonal rootstock propagation in temperate latitudes to guarantee that selected rootstocks exhibit a stable phenotypic effect. However, clonal rootstocks in Colombia are still rare in avocado producer's regions where avocados are native and seeds (as rootstocks) from creole trees are more abundant and cheaper. For this, it is imperative to evaluate which traits of the creole avocado are inherited from its open pollination half-sib seedling progenies while at the same time transferred to the scion after grafting (Cañas-Gutiérrez et al., 2022).
Gas exchange: Statistically significant differences were found for gas exchange variables. Net photosynthesis (P<2·10-16), stomatal conductance (P<2·10-16), transpiration (P<2·10-16), and efficient use of water. (P<2·10-16). Regarding A, rootstocks ID01 and ID02 presented the best net photosynthesis, expressing rates higher than 6 μmol CO2 m-2 s-1. In comparison, the accession D15 and D17 showed the lowest rates (values less than 3 μmol CO2 m-2 s-1). The average A ranged between 3 and 6 μmol CO2 m-2 s-1 (Tab. 2). For WUE variable, ID02 presented the highest value (1.70 μmol CO2/mmol H2O), followed by ID08 and ID13 (values greater than 1.40 μmol CO2/mmol H2O). The lowest WUE corresponded to ID17 (0.56 μmol CO2/mmol H2O). Regarding gs, the accession with the lowest rate was ID17 (0.011 mol H2O m-2 s-1), and the highest was for ID16 (0.15 mol H2O m-2 s-1). The highest value corresponded to ID16 (5.93 mmol H2O m-2 s-1) and the lowest to ID09 (3.35 mmol H2O m-2 s-1) for E.
Table 2. Gas exchange parameters in seventeen creole avocado rootstocks. Net photosynthesis (A), stomatal conductance (gs), transpiration rate (E), and water use efficiency (WUE).
Rootstock | A | gs | E | WUE |
---|---|---|---|---|
ID01 | 6.78±0.33 b | 0.10±0.004 fg | 4.89±0.19 bc | 1.42±0.053 bc |
ID02 | 8.31±0.30 a | 0.11±0.003 de | 4.92±0.12 b | 1.70±0.050 a |
ID03 | 5.83±0.19 cd | 0.08±0.002 hij | 4.29±0.11 ef | 1.37±0.047 bc |
ID04 | 5.90±0.18 c | 0.09±0.003 g | 4.76±0.10 bcd | 1.24±0.028 d |
ID05 | 4.99±0.17 ef | 0.11±0.004 cd | 3.73±0.09 hij | 1.37±0.054 bc |
ID06 | 4.00±0.15 gh | 0.11±0.002 cd | 4.85±0.05 bc | 0.82±0.029 f |
ID07 | 4.38±0.16 gh | 0.10±0.003 ef | 4.48±0.10 de | 0.99±0.032 e |
ID08 | 5.62±0.27 cd | 0.08±0.003 j | 4.04±0.16 fgh | 1.44±0.057 b |
ID09 | 3.13±0.16 i | 0.06±0.002 k | 3.35±0.11 k | 0.98±0.047 e |
ID10 | 5.30±0.17 de | 0.13±0.002 b | 4.50±0.07 de | 1.20±0.044 d |
ID11 | 4.47±0.18 fg | 0.11±0.003 de | 4.36±0.09 ef | 1.02±0.035 e |
ID12 | 4.51±0.12 fg | 0.09±0.003 gh | 3.53±0.08 jk | 1.30±0.035 cd |
ID13 | 5.35±0.22 cde | 0.08±0.002 ij | 3.67±0.08 ijk | 1.46±0.051 b |
ID14 | 4.42±0.26 fgh | 0.09±0.004 ghi | 4.10±0.14 fg | 1.06±0.042 e |
ID15 | 2.94±0.15 i | 0.12±0.005 bc | 4.59±0.14 cde | 0.65±0.027 g |
ID16 | 3.86±0.18 h | 0.15±0.005 a | 5.93±0.14 a | 0.65±0.025 g |
ID17 | 2.15±0.15 j | 0.011±0.04 de | 3.88±0.15 ghi | 0.56±0.038 g |
Distinct letters in the column indicate significant differences according to Tukey's test (P≤0.05). Values are means±standard error.
Mandemaker (2007) points out the importance of the avocado's physiological understanding to achieve outstanding crop yields, emphasizing the photosynthetic processes. It would allow the strategic establishment of cultivars in times or areas that allow a higher fruit yield.
CO2 fixation: CO2 fixation and stomatal conductance vary between species, presenting high, low, or null interaction. The relationship between these variables in avocado cultivation has been evaluated under the influence of different hours of the day, showing a high affinity, suggesting that stomach conductance affects photosynthetic activity in avocado leaves, therefore, its productive potential (Heath and Arpaia, 2005). The preceding explains the behavior and the evidenced relationship of the patterns ID01 and ID02, which presented the best CO2 fixation and transpiration rates, added to greater efficient water use (Tab. 2).
Dry matter: The accumulation of dry matter presented significant differences between the creole avocado accessions for leaves (P=1.67·10-05), stem (P=4.46·10-08), roots (P=3.24·10-09), seeds (P=1.28·10-10), and whole plant (P=8.18·10-05). The greater dry matter accumulation according to each organ was found for leaves (ID11, ID-03, ID07, ID14, ID15, ID08), stem (ID08, ID15, D16), roots (ID08, ID14, ID16), and seeds (ID01, ID02 ID07, ID13). For the whole plant, ID01, ID08, and ID15 were the ones that presented a greater accumulation at 90 d of development (the time in which it is considered that the plant obtains the optimal stage for the grafting process) (Tab. 3).
Table 3. Leaf dry matter (LDM), rootstock stem dry matter (SDM), root dry matter (RDM), seed dry matter (SeDM), and total plant (TDM) dry matter in seventeen creole avocado rootstock (RS) (values expressed in grams).
RS | LDM | SDM | RDM | SeDM | TDM |
---|---|---|---|---|---|
ID01 | 8.84±0.28 efg | 5.08±0.57 cde | 4.96±0.46 ef | 24.63±0.70 a | 43.50±0.11 a |
ID02 | 7.51±1.04 g | 5.96±0.24 bcd | 4.76±0.92 f | 16.51±1.55 b | 34.73±0.26 bcd |
ID03 | 12.73±1.00 abcd | 4.07±0.55 ef | 7.45±0.59 cd | 9.78±1.18 efgh | 34.02±0.20 cde |
ID04 | 10.84±0.51 cdef | 4.69±0.01 def | 7.52±0.27 cd | 9.18±0.02 fghi | 32.23±0.00 cdef |
ID05 | 7.18±0.44 g | 3.65±0.03 f | 4.90±0.03 ef | 7.00±0.69 hij | 22.72±0.15 g |
ID06 | 8.27±0.07 fg | 4.17±0.26 ef | 4.63±0.47 f | 9.13±0.69 fghi | 26.19±0.14 efg |
ID07 | 12.97±0.15 abc | 3.76±0.10 f | 7.62±0.55 cd | 14.90±1.11 bc | 39.24±0.11 abc |
ID08 | 14.54±0.98 a | 7.23±0.28 ab | 9.51±0.36 ab | 11.64±1.04 cdef | 42.91±0.17 a |
ID09 | 8.60±0.92 efg | 3.98±0.38 ef | 4.93±0.45 ef | 7.15±0.04 ghij | 24.65±0.21 fg |
ID10 | 9.89±0.61 defg | 4.47±0.76 ef | 5.28±0.95 ef | 10.74±0.53 defg | 30.38±0.01 defg |
ID11 | 12.06±1.81 abcd | 4.61±0.68 ef | 6.68±1.41 de | 10.19±1.59 efgh | 33.53±0.27 cde |
ID12 | 10.77±0.52 cdef | 4.54±0.24 ef | 6.03±0.52 def | 13.17±3.05 bcde | 34.50±0.52 cd |
ID13 | 8.88±0.93 efg | 2.12±0.74 eg | 4.57±0.03 f | 14.30±0.23 bcd | 29.86±0.04 defg |
ID14 | 12.85±1.55 abc | 4.62±0.43 ef | 10.53±0.01 a | 5.59±0.05 ij | 33.59±0.01 cde |
ID15 | 13.82±0.34 ab | 7.68±0.20 a | 8.58±0.55 bc | 12.73±0.89 cdef | 42.80±0.014 ab |
ID16 | 11.22±0.20 bcde | 6.03±0.20 bc | 11.37±0.87 a | 4.76 ±0.29 j | 33.37±0.05 cde |
ID17 | 8.54±1.12 efg | 4.83±0.66 cdef | 5.95±0.77 def | 9.72±0.43 efgh | 29.03±0.08 defg |
Data are means±standard error (n=20). Different letters in the same column denote significant differences among rootstocks by one-way ANOVA after LSD Test (P<0.05).
The adequate distribution of the photo assimilated obtained from photosynthesis to the different organs is essential for the yield of any productive system since the yield is limited by the potential for the accumulation of biomass in organs of interest (Peil and Galvez, 2005). Generally, the first plant growth stage, from greater to lesser biomass accumulation, occurs in leaves, stems, fruits, and roots (Hernández et al., 2009; Betancourt and Pierre, 2013). Peil and Galvez (2005) indicate that the variation in dry biomass partition and distribution during plant development causes a decrease in yield and affects the quality of the fruits. According to the above, the rootstocks that presented the highest total biomass accumulation were ID01, ID08, and ID15. This parameter allows inferring the assimilation capacity of the accessions and their future productive potential. Even so, a material that presents a high accumulation of dry matter in the seed will allow better storage of the nutrients necessary to develop both the rootstock and the scion (Mejía-Jaramillo et al., 2022). It is possible to infer that this characteristic will allow a better performance of the cultivar in its production stage. In the stages of greater susceptibility, there is a greater reservoir of elements, the most outstanding being materials D01, D02, D07, and D13.
Plant growth: Statistically significant differences were found for the variables: rootstock stem diameter (P=0.00102), rootstock height (P<2·10-16), leaves number (P=1.85·10-06), leaf area (P=2.4·10-05), leaf area index (P=4.24·10-07) and specific leaf area (P=0.0103). All the accessions presented diameters between 6 and 8 mm at the time of grafting. However, the ID08 and ID16 genotypes stood out from the other materials since they registered diameter values greater than 9 mm. Regarding rootstock height, the highest values were shown by ID01 and ID15, exceeding 60 cm in height; ID07 and ID14 recorded a lower plant height at the time of grafting. The highest leaves number corresponded to accessions ID08 and ID15, which had the largest leaf area. Accessions ID06 and ID17 achieved the highest values for the specific leaf area, and materials ID04, ID07, and ID14 had the lowest SLA records (Fig. 1).
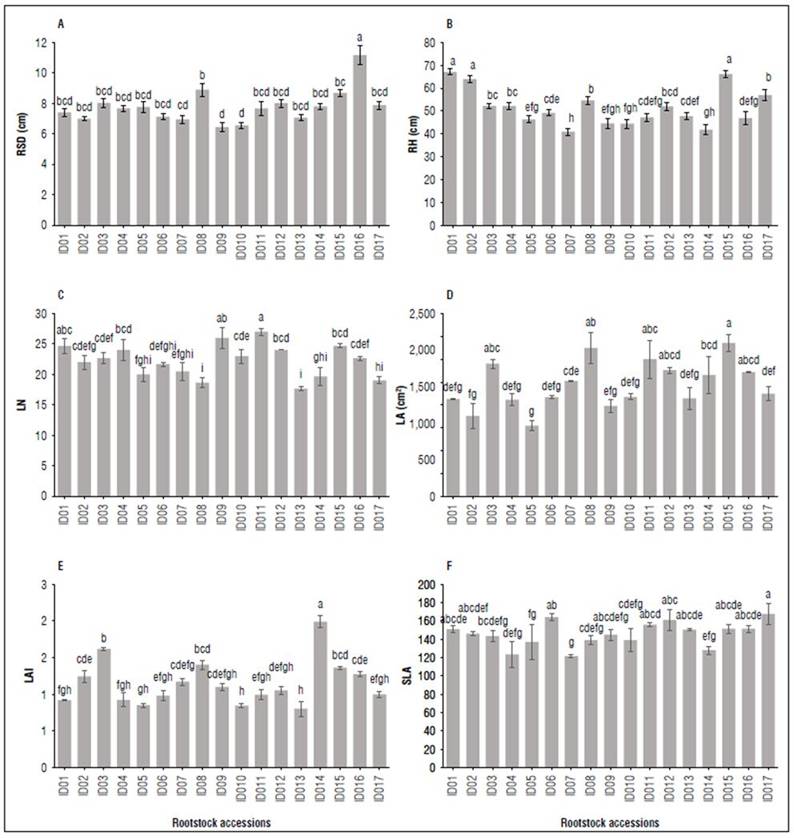
Figure 1. Vegetative growth: RSD: Rootstock stem diameter (A), RH: Rootstock height (B), LN: Leave number (C), LA: Leaf area (D); LAI: Leaf area index (E) and SLA: Specific leaf area (G) of seventeen creole avocado rootstocks age of three months after planting. Different letters on bars corresponding to the same response variable indicate significant differences among rootstocks according to Tukey test (P< 0.05). Vertical bars correspond to standard error. Rootstocks n=20.
Growth is the response of the genotype to environmental conditions and their interaction, which has been quantified through parameters that describe both changes in shape and size. This quantification has been based on adjusting classical and functional mathematical expressions to simple determinations such as the change in leaf area, height, diameter, and weight accumulation, among others (Hunt, 2003). These have made it possible to indirectly characterize the physiological efficiency of the plant and its ability to produce marked morphological changes in response to the environment (Paine et al., 2012; Gonçalves et al., 2013).
It is known that environmental factors directly influence the growth of plants and determine the degree to which they express their genetic potential, which is essential for the selection of rootstocks. The rootstock selection should be directed towards productive, vigorous, and healthy trees, mainly from creole genotypes that express desirable agronomic characteristics (Lazare et al., 2021). Therefore, evaluating quantitative parameters as a selection criterion in a genetic improvement program is essential since the search will focus on individuals who best behave in each environment of their origin. Thus, selecting a suitable rootstock must be based on the environment or agroclimatic zone in which the grafted tree will be cultivated. In other words, due to a triple rootstock/scion/environment interaction, rootstock selection from creole seedling genotypes is challenging (Cañas-Gutiérrez et al., 2022).
These behaviors are attributable to the genotype and the edaphoclimatic conditions they interact with since both factors influence plant development. Specific parameters are considered selection criteria in those materials that express desirable characteristics in the shortest possible time; therefore, early identification of avocado genotypes with potential for use as rootstock is desirable (Barrientos-Priego, 2017; Viera et al., 2017). In this sense, it is sought that the rootstocks have a minimum grafting caliber of 5 mm, foliage with good development in terms of number and leaf area, leaves with thick texture, and higher rootstock heights at 40 cm (Viera et al., 2017). They are creole or local seed trees that have best demonstrated their attributes. According to these parameters, the present investigation showed diameters between 6 and 8 mm at the time of grafting, with the ID08 and ID16 genotypes standing out (> 9 mm). Regarding the other parameters, such as height (> 60 cm), leaf number, leaf area, and specific leaf area, materials such as ID01 and ID15, ID08 and ID15, ID08 and ID15, and ID06 and ID17 stood out, respectively, for each indicated variable.
Physiological parameters provide characteristics that would enable understanding the adaptation of the genotype to different altitude levels (Halbritter et al., 2018). In this sense, the behavior of ID08 stands out, even though its photosynthetic rate is not the highest respect to the other materials. The genotype presented one of the largest diameters (> 9 mm) at the time of grafting, which allows for inferring its capacity in terms of the distribution and partitioning of the assimilated.
Principal components and cluster: According to the analysis carried out (Fig. 2), it is observed that the variable weight (g) gives the highest percentage in terms of the principal components (55.3%), referring to the physiological variables, where the first component (Dim1) shows the (30%), while the second principal component (Dim2) presented the (25.3%) of the variance represented by the variables, for the seventeen genotypes. In addition to the above, the variables that showed the greatest positive contribution were A, E, and gs, with the ID16 and ID09 genotypes registering the highest values. On the contrary, the ID09 genotype was the one that recorded the lowest value.
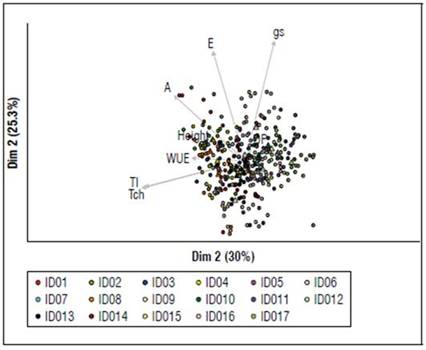
Figure 2. Correlation between quantitative descriptors and the first two principal components, CP1 and CP2, for the seventeen creole avocado rootstocks. A: net photosynthesis, E: transpiration rate, gs: stomatal conductance, WUE: water use efficiency, Tch: air temperature, Tl: Leaf temperature, DP: rootstock diameter.
For WUE, leaf temperature (Tl), and chamber temperature (Tch) (the last two highly correlated), the highest values were shown by ID01, ID02, ID03, ID04, ID08, and ID09. The lowest values corresponded to ID05, ID10, ID11, ID12, ID15, ID16, ID17. Regarding rootstock height and rootstock diameter, these variables contribute little to the variance of the individuals; this is possibly due to the phenological state in which they are found.
The hierarchical clustering method allowed the identification of three groups. Group 1 is made up of thirteen accessions (ID03, ID04, ID05, ID06, ID07, ID08, ID09, ID11, ID12, ID13, ID14, ID16, and ID17) who share the departments of Antioquia and Caldas, as their origin. These accessions come from life zones such asvwf-P, wf-PM, vwf-T, and wf-LM with photosynthetic rates between 3.13±0.16 and 5.90±0.18 μmol CO2 m-2 s-1. Group 2 is formed by genotype ID10 (Department of Caldas with life zone vwf-PM) with net photosynthesis rates of 5.30±0.17 μmol CO2 m-2 s-1. Finally, group 3 comprises three accessions (ID01, ID02, and ID15) from the Department of Antioquia (vwf-T, vwf-PM, and vwf-PM life zones) with net photosynthetic rates between 7.51±1.04 to 13.82±0.34 μmol CO2 m-2 s-1 (Fig. 3)
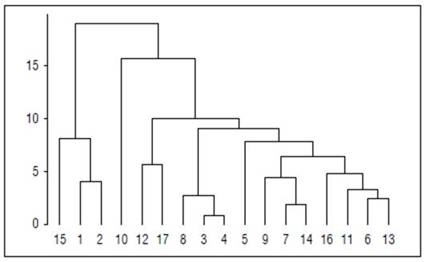
Figure 3. Euclidean distance-based dendrogram for seventeen avocado rootstocks genotypes using quantitative descriptors.
Genetic variability is the totality of the variants that a species represents. This variation can be expressed through phenotypic and genetic characteristics (Franco and Hidalgo, 2003). It can be attributed to the dynamics of the interaction between biotic and abiotic factors where the plant develops, which generates high diversity in the genetic information of populations or species, which is transmitted over time to future generations (Hernández-Villarreal, 2013). The characterization descriptors are an easy and fast tool for selecting phenotypes. However, they generally represent characters with high heritability and easy detection, which can be expressed similarly in almost all the environments under which the individuals are established, also allowing a selection of those with better characteristics for certain avocado producers (Hernández-Villarreal, 2013).
The above facilitates the identification of the study population's genetic variability to determine the best descriptors (discriminants) to evaluate in a population (Hernández-Villarreal, 2013). Knowledge of the genetic diversity of avocados has been carried out using different techniques, including molecular tests (Amplified Fragment Length Polymorphism - AFLP) and characterizations of morphological variables, which have resulted in the identification of similar clusters by both techniques. This has allowed relation to the differences observed, generally due to the geographical origin of the materials evaluated (Cañas-Gutiérrez et al., 2015). As evidenced in the hierarchical grouping analysis carried out because of the present research, which allowed the identification of three groups. Group 1 is made up of thirteen accessions (ID03, ID04, ID05, ID06, ID07, ID08, ID09, ID11, ID12, ID13, ID14, ID16, and ID17), group 2 for ID10, and group 3 by ID01, ID02, and ID15.
The avocado is divided into three ecological races (Mexican, Antillean, and Guatemalan) with a similar genome (2n=24) (Sánchez-González et al., 2020; Reyes-Herrera et al., 2020; Lazare et al., 2021). Their hybridization quickly occurs, considering that the hybrids obtain advantages in adaptation to climate and better agronomic characteristics (López-Galé et al., 2022). Therefore, the clustering method can originate similar avocado echo types in terms of interaction with the environment due to the similarity of the environmental conditions of its area of origin. Hence, attributing specific adaptations are observed in the different accessions as evidenced in the analysis of hierarchical grouping, which generated 3 clusters of accessions from similar morphological variables and a cluster according to the influence of the various principal components. Because the avocado presents an open pollination, it has great genetic variability allowing a substantial use of its attributes (Peng et al., 2013).
Cañas-Gutiérrez et al. (2022) indicate that elite creole “plus trees” may serve as promissory donor seedling rootstocks for avocado cv. Hass orchards due to the inheritance of their outstanding trait values. This reinforces the feasibility of leveraging natural variation from “plus trees” via open-pollination half-sib seedling rootstock families.