INTRODUCTION
A set of strategies to mitigate food insecurity through agrobiology is currently being implemented worldwide with some success. These strategies include biocontrol, biofertilization, and biostimulation technologies, which have shown potential to mitigate the difficulties associated with agricultural practices, especially under limiting conditions in aridisols (Reeves et al., 2016; FAO, 2022; Li et al., 2022). Biostimulation is based on the use of substances or microorganisms that, when applied to plants, trigger physiological responses that lead to improvements in nutritional efficiency, tolerance to biotic and abiotic stress (Van Oosten et al., 2017), stimulate the metabolism, and generally favor the performance of crop plants (Nardi et al., 2016). Biostimulants include inputs based on microorganisms, fractions of humified organic matter, hydrolysates of proteins, amino acids and other nitrogenous compounds (Nardi et al., 2016), oligosaccharides, inorganic compounds, green algae extracts, and botanicals (Du Jardin, 2015).
On the other hand, humic acids are a fraction of humified organic matter, whose supramolecular structure is related to its bioactivity and determines its biostimulatory action. This comprises a series of physiological effects that lead to proliferation, elongation, and modifications in the root architecture, increase in the absorption and transport of ions, efficiency of primary metabolism (Aguiar et al., 2018), regulation of molecular processes, and induction of secondary metabolites synthesis (Shah et al., 2018), as well as systemic responses to stressors (da Piedade Melo et al., 2017) thanks to a "priming effect"(Canellas et al., 2020). Moreover, they also induce the increase in the production of exudates (Canellas et al., 2019), which affect the magnitude and activity of the rhizosphere microbial community, plant-microorganism communication, therefore, the establishment and performance of symbiotic, associative and endophytic interactions by plant growth-promoting microorganisms (Roomi et al., 2018; Bulgari et al., 2019; García et al., 2019; Nunes et al., 2019; Jindo et al., 2020).
The intensification of organic agriculture in arid and semiarid zones require to adapt cultivars tolerant to adverse climatic and ecological conditions, such as high temperatures, drought and salinity; thus, it is convenient to use biostimulating agents, such as humic acids, that relieve stress and promote plant growth and development. The genus Bacillus comprises a wide variety of bacterial plant growth-promoting species that express several biostimulation mechanisms, including indole acetic acid (IAA) synthesis (Tejera-Hernández et al., 2011; Rojas, et al., 2013). In addition, characteristics such as their persistence in a broad spectrum of environmental conditions, metabolic versatility, presence of resistance structures as endospores, and the motility that facilitates plant colonization, make genus Bacillus very useful in agricultural biotechnology (Tejera-Hernández et al., 2011), Such advantages acquire importance in arid and semi-arid soils compared to other microorganisms commonly used as bioinoculants for agriculture. Their role has been documented as an adjuvant for the soil rehabilitation, and the adaptation of pioneering plants in desert areas in a joint application with organic amendments (Bashan et al., 2012).
The chemical composition of humic acids varies according to their source; thus, certain supramolecular characteristics are linked to variations in their bioactivity. Bioactivity patterns associated with specific structural configurations have been previously described. For instance, labile and functionalized humic fractions are related to root proliferation, whereas less functionalized and more recalcitrant fractions are associated with root elongation (García et al., 2016a). However, these patterns are not regular, and the bioactivity of humic acids concerning their supramolecular structure is still a subject of discussion (García et al., 2019).
Variable results have been observed in the joint application of humic acids and plant growth promoting rhizobacteria (PGPR). Eventually, this combination could have not the best effect on plant performance compared to the separate application of each biostimulant (Ahmad et al., 2016; Valero et al., 2016; Bacilio et al., 2017; Bratkova et al., 2021). Some authors have hypothesized that these results are generated by modulating effects of a “hormonal balance” in microorganism-plant communication (Blouin, 2018). Therefore, it is important to evaluate the bioactivity of humic acids whose chemical nature varies according to their origin, as well as the effects of their interaction with PGPR.
This work aims to evaluate the relationship between the bioactivity and the structural characteristics of humic acids obtained from two locally available raw materials in the semi-arid La Guajira peninsula, Colombia: vermicompost made with goat manure -goats are the main livestock activity in the region- and the lignite coal generated as a by-product of open-pit coal mining -it is also a large-scale economic activity in the area. Additionally, we sought to analyze the effect of treating maize plants, under controlled conditions and field conditions, with each humic acid separately and together with the strain Bacillus mycoides BSC25. The latter has been evaluated previously due to its performance as a PGPR under the limiting conditions that derive from the aridity of this territory (Valero-Valero et al., 2021; Beleño-Carrillo et al., 2022).
MATERIALS AND METHODS
Extraction of HA
Two raw materials common in La Guajira peninsula were used as humic acids (HA) source: a lignite generated as a by-product of coal mining, and goat manure vermicompost. 1 kg of each material was taken and oven-dried at 70°C until to obtain constant weight. Subsequently, the total humic extract was obtained by applying the classic extraction method with alkaline solutions (1N NaOH), following the standard methodology described by the International Humic Substances Society for each type of sample (Chang et al., 2014). The organic matter was extracted from the samples with 1 N NaOH (10 h, 60°C) and subsequently, the extract obtained was allowed to stand for 24 h and was filtered using Whatman paper No. 40 to separate the humic and fulvic acids from the insoluble residue or humins. The HAs in the extracts were separated from the fulvic acids (FAs) by acidifying the solution to pH 2 using 1N HCl. This fraction was collected and taken to successive washes with distilled water and subsequent centrifugation; then, the HA extract was freeze dried and stored in plastic tubes at -4°C.
Chemical nature of HA
The chemical characterization of the two AH types (HAC: humic acid-coal; HAVC: humic acid-vermicompost) comprised: 1) elemental composition (C, H, O, N, S) using ASTM D5373 with an EAI CE-440 Elemental Analyzer (Pantoja-Guerra and Valero-Valero, 2020); 2) analysis through Fourier transform- Infrared spectroscopy (FT-IR), the protocol described by Spaccini and Piccolo was followed to obtain the IR spectrum of each HA sample (Spaccini and Piccolo, 2009); and 3) nuclear magnetic resonance analysis (13C-CP-TOSS (1H)-NMR), NMR spectroscopy was performed in solid state. As proposed by Zhang et al. (2017), samples were processed on a Bruker Avance III HD Ascend 400 MHz (9.4 T) spectrometer. For the interpretation of the spectra, the chemical shift recorded between 0 and 200 ppm was divided into the following resonance regions: C-alkyl (0-45 ppm), C-methoxy and N-C (45-60 ppm), C-O-alkyl (60-110 ppm), C-aromatic (110-160 ppm), C-carboxy and carbonyl, aldehydes, and ketones (160-200 ppm) (Spaccini and Piccolo, 2009). The integration of the area under the curve was subsequently performed at each range. In this way, quantitative data of the relative percentage of 13C in each group were obtained. Finally, the aromaticity index of each HA sample was determined with the quantitative data (Spaccini and Piccolo, 2009).
Evaluation of humic acid bioactivity in plant tissue
HA bioactivity was assessed by the coleoptile elongation test (Valero et al., 2018a). Corn seeds (variety ICA-109) were germinated on agar-agar plates at 28°C. Once the coleoptiles emerged, 5 mm segments were cut from the base. The segments were immersed in Petri dishes with 10 mL of HAC or HAVC solutions in 2 mM CaCl2 at concentrations of 10, 25, 50, 75, 100, 150, and 200 mg carbon per liter of solution (mg C L-1). As a control treatment, 2 mM CaCl2 solution was used, and 10 repetitions were made per treatment. The coleoptiles were shaken in the dark in the different solutions for 48 h at 150 rpm.
After the stirring period, the coleoptiles were photographed with a digital camera next to a pattern of known length. The change in the length of the coleoptiles was determined by digital image analysis by the ImageJ software, Fiji version.
Association between the chemical structure of HA and their bioactivity
The coleoptile length data, the elemental composition of HA, and their chemical composition by functional groups (13C-CP-TOSS (1H)-NMR) were analyzed by principal component analysis (PCA) multivariate statistics using the PAST statistical package (v. 4) to compare the bioactivity of both types of AH and find a possible association between the bioactivity and the chemical nature of their structure.
Effect of humic acid application and inoculation of B. mycoides BSC25 on corn plant growth
Growth chamber tests
Corn seeds, variety ICA-109, were disinfected in a 2.5% NaClO solution for 1 min; then, they were rinsed 5 times with sterile distilled water. Subsequently, they were placed in a humidity chamber, and after 4 d of germination they were transferred to pots with 300 g of soil obtained from a semi-arid area in the Alta Guajira (municipality of Uribia: 11°35’38.8’’ N and 072°19’27.9’’ W). The soil characteristics are presented in table 1.
Table 1. Main characteristics of the soil used for growth chamber and field tests.
Corn crop | Texture | pH | OM | Ca | Mg | K | Na | CEC | P | S | Fe | Mn | Cu | Zn | B | N | |||
---|---|---|---|---|---|---|---|---|---|---|---|---|---|---|---|---|---|---|---|
Sand % | Silt % | Clay % | Class | % | cmolc kg-1 | mg kg-1 | % | ||||||||||||
78 | 18 | 4 | AF | 7.9 | 0.84 | 7.4 | 1.3 | 6.7 | 0.12 | 9.5 | 127 | 9 | 7 | 3 | 1 | 2 | 1 | 0.06 |
OM: Organic matter
Six treatments were applied under a completely randomized experiment: HAC, HAVC, Bacillus mycoides BSC25 (Bm) (Valero et al. 2014) HAC+Bm, HAVC+Bm, and control. Each treatment had five replicates. The treatments were applied at two times: 1) at planting time seedlings, in the pots; 2) 15 d after the first application. The applied Bm doses were 10 mL of bacterial suspension at 1·108 CFU concentration applied directly to the root. For the HA treatment, 5 mL doses were applied by foliar spray at a concentration of 100 mg C L-1, in 2 mM CaCl2 solution.
The plants were kept in a growth chamber under the following conditions: photoperiod 12 h, relative humidity near to 60-70%, and temperature between 28 and 30°C. Irrigation was done with sterile distilled water every 2 d. After 28 d, the plants were harvested, taking care not to damage the roots. Plants were washed and dried for 72 h at 65°C; then, the dry weight was determined; the root and shoot were weighed separately.
Field test
The seeds were disinfected and germinated in a humidity chamber as explained above. This experiment was carried out under a completely randomized design. For this, rows of 12×1 m were built. The plants were separated 80 cm from each other. The assay consisted of 6 treatments: HAC, HAVC, Bm, HAC+Bm, HAVC+Bm, control (distilled water). Three replicates per treatment were evaluated, each one consisting of a group of 8 plants. Sowing and other crop activities were carried out according to the tradition of local producers, without agrochemical application.
The crop beds were prepared and the soil was tilled, the seeds were placed in germinators with forest soil saturated with water. One week after seedlings emergence, a 4 mL dose of HAC or HAVC solution at 100 mg C L-1 was applied to the plants according to each treatment. After 12 d, eight plants were transplanted from the germinators to the soil; 2 d later, a second 30 mL dose of the HA solution, or 30 mL of Bm suspension were applied to the corresponding treatments. When flowering began, a last dose of each treatment was applied. It was monitored for 82 d, observing developmental variables such as number of fruits per plant. Eighty-two days later planting, two plants were harvested randomly from each repetition of each treatment, carefully avoiding damaging the roots. They were washed carefully; then, dry weight of roots and shoot were determined.
RESULTS AND DISCUSSION
Extraction and characteristics of HA
The extraction yield of total humic extract (THE) was 44.68±7.3% for coal and 32.31±7.5% for vermicompost; however, the percentage of the fraction of HA that can be separated from the THE of the two raw materials is similar: 7.39±1.1 and 6.26±0.96% for coal and compost, respectively.
This result can be explained because coal undergoes a geochemical process of transformation of organic matter from plant debris. It results in a highly condensed material (Giannouli et al., 2009), where most of the original plant biomass was transformed to constitute the mobile fraction (humic substances) or the fixed fraction (carbonaceous matrix) without residues of other types of molecules originally present in the plant from which the carbonification process took place. The compost, in turn, is obtained by a plant debris biological transformation process, not geochemical. This process occurs in a very short time scale, giving rise to humic substances by condensation and microbial polymerization of organic matter. Thus, together with the humified organic matter, another type of molecule is generated and is the result of the partial transformation of the original plant materials (Canellas et al., 2010).
Figure 1 shows the elemental composition of the two types of HA -both have low C and N content-, the differences are mainly due to a high oxygen content in the HAC and a higher carbon content in the HAVC. The higher oxygen content in the HACs is an outstanding feature that could indicate a higher content of carbonyl, carboxyl, and hydroxyl functional groups. It could confer greater reactivity to the HA supramolecule, as previously explained (Muscolo et al., 2007a; Muscolo et al., 2013; Scaglia et al., 2016; Fischer 2017). VCHA had a higher carbon content, which can be explained by a higher content of aliphatic structures and carbohydrates (Nebbioso and Piccolo, 2011), as evidenced in the 13C-CP-TOSS (1H)-NMR analysis (Fig. 2).
The semi-quantitative determination of the 6 types of major functional groups detected by 13C-CP-TOSS(1H)-NMR analysis (Fig. 2) revealed that the two types of HA have a high predominance of aromatic fractions; however, HAC presents a higher value. This result is consistent with the geochemical process of coalification that increases the degree of aromatic condensation (Ritchie and Perdue, 2008; García et al., 2016b). Aliphatic carbon is the following fraction in relative abundance; HAVC exhibit the highest content. This may be related to abundant carbohydrate residues due to the predominant plant residues in the manure used as raw material to obtain the vermicompost (Cavagnaro, 2014; García et al., 2016b). Carboxylic and carbonylic carbon are almost twice as high in HACs, which is related to the high oxygen content observed in the elemental analysis. Similarly, the carbon is much higher in aldehydes and ketones, while the carbon in polysaccharides, alcohols and amino sugars is higher in HAVC. The carbon in methoxy and N-alkyl groups has similar values in both types of HA, which corresponds to similar values of the nitrogen content in them (Fig. 2).
From the above, we can infer a more condensed character together with the value of the aromaticity index, with high content of oxygen functional groups in HACs, and a more aliphatic character in HAVCs with presence of simpler polysaccharides. The high carboxylic content in HACs and the aliphatic character in HAVCs have been previously related to their degree of bioactivity (Valero et al., 2018a). The molecular characteristics described show resonance patterns like those observed in other HAs with high bioactivity expressed in the stimulation of H+ATPase pumps and the proliferation of secondary roots (Canellas et al., 2002; 2010; García et al., 2016a). This effect has been associated with well-defined resonance signals around 56 (C-methoxy and N-C), 125-150 (aromatic), and 175 ppm (C-aldehydes, ketones and carboxy) (Aguiar et al., 2013; García et al., 2019).
Figure 3 shows the spectra generated by the two types of HA by FTIR analysis. In general, their profile agrees with the characteristics of the functional groups typical of the humified organic matter(Canellas et al., 2010; Sharma et al., 2016). It reflects a consistent quality parameter described by the presence of a broad band around 3,400 cm-1, characteristic of OH groups in alcohols, free phenols, and carboxylic groups; a subtle narrowing band around 2,920 cm-1; and a bending band towards 1,380 cm-1. Together, these correspond to the C-H bond in aliphatic groups; a narrowing band corresponding to the C=O bond around 1,700 cm-1; a narrowing band at 1,717 cm-1 corresponding to aromatic rings; and a bending band corresponding to OH or to the C=O narrowing around 1,280 cm-1 (Valero et al., 2018b). The only appreciable difference between the two types of HA corresponds to two bands of moderate intensity around 1,100 cm-1 in the HAC spectrum that can be given by the presence of alkyl C-O groups and indicate that these are richer in carbohydrate fractions (Valero et al, 2018b).
Humic acid bioactivity
Bioactivity refers to some hormonal effects of HA on plants, which activate biochemical mechanisms that modulate plant growth (Nardi, et al., 2021). These mechanisms have not yet been fully elucidated (García et al., 2019); however, they have been partially characterized through morphological, histological, biochemical, and transcriptomic approaches. Auxin-like effect is the hormonal mechanism of humified organic matter with more available evidence (Muscolo et al., 1999; Canellas et al., 2002; Nardi et al., 2002; Muscolo et al., 2007b; Trevisan et al., 2010; Canellas et al., 2011; Canellas and Olivares, 2014; Mora et al., 2014; Nardi et al., 2018; Canellas et al., 2020).
Table 2 presents the effect of the application of both types of HA on the coleoptiles corn elongation. A bioactivity pattern is observed according to the origin of the samples, it is also associated with the structure of the HA. HAC exhibited a coleoptile elongation effect at low concentrations (25-50 mg L-1), while HAVCs exhibited coleoptile elongation ability at higher concentrations (100-200 mg L-1).
Table 2. HA bioactivity by the coleoptile elongation test.
HACs exhibited higher bioactivity, since lower concentrations were required to cause elongation of the coleoptiles. 13C-CP-TOSS-NMR and FTIR analyses indicate that these HAs have a higher content of aromatic carbon, carboxylic, and carbonyls. This is consistent with the elemental composition analysis that showed a higher oxygen content (Fig. 1) and indicates greater bioactivity (Muscolo et al., 2020). Some polar HA small fractions occluded in hydrophobic polyaromatic domains can disaggregate themselves from their suprastructure, cross some plant tissues, interact with the cell membrane through hydrogen bonds, and enter the cell (García et al., 2019). Both the intracellular access of HA fractions, as well as adsorption interactions with the membrane, trigger hormonal effects in plant tissues (Ojwang’ and Cook 2013; García et al., 2014). The presence of IAA and other molecules with auxin-like activity within the humic suprastructure has been widely reported (Canellas et al., 2002; Valero et al., 2018b; Pizzeghello et al., 2020).
The highest concentrations of HAVC were found to stimulate the elongation of the coleoptiles. García et al. (2019) suggested that HAs rich in aromatic and aliphatic (recalcitrant) structures require higher concentrations for effective plant growth stimulation. Despite HAC having a slightly higher aromatic content, HAVCs exhibited a significantly higher aliphatic content (Fig. 2). This observation is consistent with the overall higher total carbon content found in HAVC. Notably, these results deviate from the typical behavior of this type of HA (Ait Baddi et al., 2003), possibly due to the high lignin content of the composted feedstock.
The vermicompost came from goat manure and the tropical dry forest litter in the middle Guajira, this includes woody tree species such as Aspidosperma polyneuron, Platymiscium pinnatum, Tabebuia billbergii, among others (Gualdrón, 2011). Moreover, the diet of the goats in this area is mainly based on low-growing semi-woody plant species such as Tabebuia billbergii, Mimosa arenosa, and Bignoniaceae (Gualdrón, 2011). Therefore, the advanced stage of lignification in the composted raw material is a plausible explanation for the high aromatic content of HA.
Principal component analyses (Fig. 4 and 5) make it possible to better explain these inferences. The bioactivity of HACs, expressed as coleoptile elongation, is observed in low concentrations (10-25 mg C L-1) and is associated with the aromatic, carboxylic, carbonyl, and oxygen content in their supramolecular structure. In contrast, HAVC bioactivity is expressed at high concentrations (100-200 mg C L-1) and can be explained by its content of aliphatic carbon, alkyl, and carbohydrates.

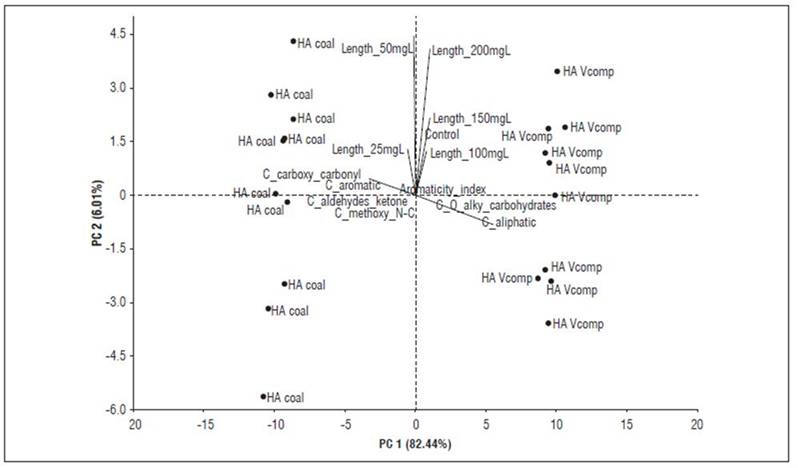
Figure 4. PCA showing the relationship between the data resulting from the quantification of carbon types based on the 13C-CP/MAS-NMR spectrum of HAC and HAVC, and the bioactivity (elongation coleoptile test).
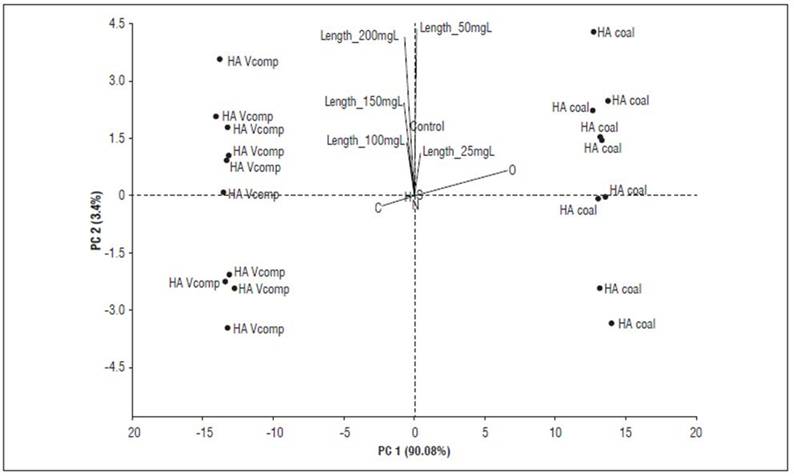
Figure 5. PCA showing the relationship between the data resulting from the elemental analysis of HAC and HAVC, and the bioactivity (elongation coleoptile test).
These bioactivity patterns associated with chemical characteristics of HAs, as well as the origin of the samples, are consistent with previous reports (García et al., 2016a, 2016b; García et al., 2018; García et al., 2019; Araújo et al., 2021). However, it is unlikely that fixed and predictive molecular patterns of bioactivity can be defined; on one hand because the bioactivity mechanisms of HA have not been fully elucidated (García et al., 2019; Nardi et al., 2021); on the other hand, the high variability in the sources of HAs makes it difficult to predict their bioactivity. In this case, the type of composted organic matter gave rise to HAs particularly rich in aromatic and aliphatic carbon.
Effect of HAC/HAVC application and inoculation of B. mycoides BSC25 on maize plants growth
Figure 6 shows the HAC and HACV application and inoculation with B. mycoides BSC25 results under growth chamber and field conditions. The treatments led to different results in foliar biomass and root biomass.
In the growth chamber assay, all treatments except HAC induced increases in foliar biomass. The contact type of HAs with plant tissues is different depending on whether they are applied at the shoot or root level. At the foliar level, the entry of small molecules derived from the humic superstructure is unlikely; therefore, bioactivity will depend on the contact of the molecules’ surface with the cells of the leaf tissues (García et al., 2019; Nardi, et al., 2021). So, it is reasonable to assume that HA with high content of alkyl carbon and carbohydrates -which are more reactive (García et al., 2016b; Nardi et al., 2021)- better stimulate the biomass of the leaves when applied by leaf spraying. This bioactivity type may be attributed to several types of like-hormone mechanisms, such as auxins (Canellas et al., 2002; Trevisan et al., 2010; Canellas et al., 2011; Nardi et al., 2018; Canellas et al., 2020), cytokinins (Pizzeghello et al., 2013; Mora et al., 2014), abscisic acid-ABA (Olaetxea et al., 2019; Canellas et al., 2020), alkamydes (Zandonadi et al., 2019), NO (Mora et al., 2014), gibberellic acid (de Sanfilippo et al., 1990; Nardi et al., 2002; Canellas et al., 2020), and biotic and abiotic stress response enzymes involved in the homeostasis of reactive oxygen species (catalases, superoxide dismutase, and peroxidases) (García, et al., 2016a; García et al., 2019; Canellas et al., 2020).
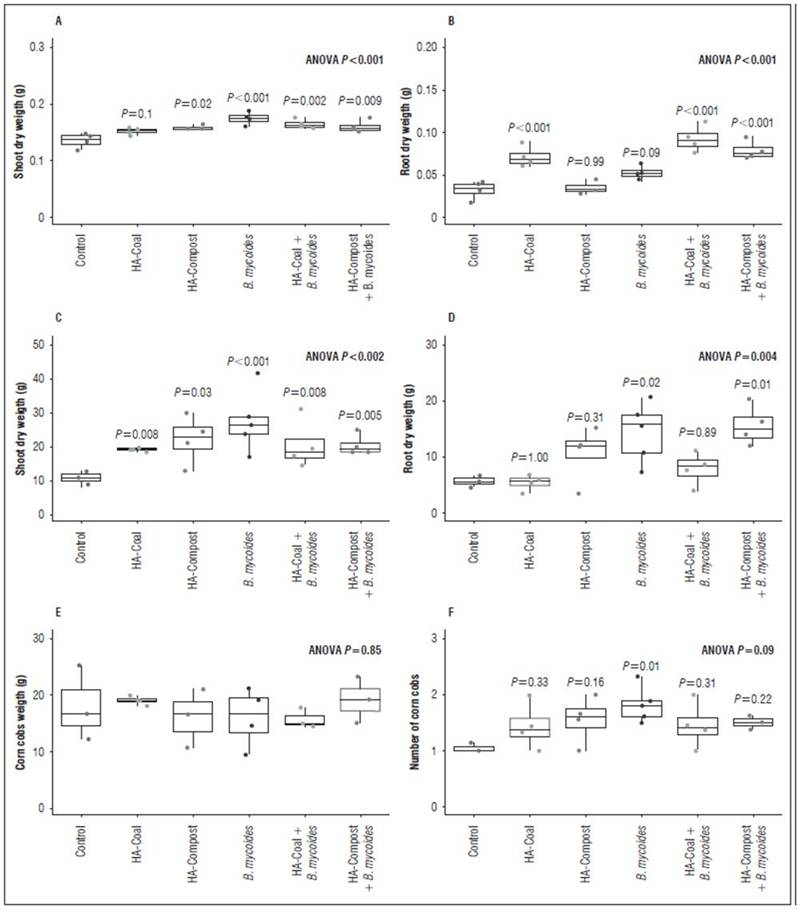
Figure 6. Effect of applying HAs and inoculating B. mycoides BSC25 on maize plants, in the growth chamber and in the field.
On the other hand, in this same test (growth chamber), all treatments except HAVC showed increases in root biomass. Although the application of HA was done in a foliar manner, root stimulation was also observed, as has been previously described (Canellas and Olivares, 2014; Canellas et al., 2020). This occurs because HA stimulation at the foliar level also triggers signaling and transcriptional responses involving root development such as auxin response factors (ARF) (Elmongy et al., 2020), ABCB polar auxin transporters (Tahiri et al., 2016), calcium-dependent membrane receptors (Canellas et al., 2020), small auxin up-regulated RNA (SAUR) genes (Canellas et al., 2020), and H+ATPase. This evidence suggests that HA stimulation at the root level is primarily due to auxin-like effects (Elmongy et al., 2020; Araújo et al., 2021). This is consistent with the results obtained in this work (Tab. 2); the HAC sample, which caused increases in root biomass, was rich in oxygen, carboxylic, and aromatic groups (Fig. 4 and 5). These chemical characteristics have been associated with auxin-like effects (García et al., 2016a; García et al., 2019).
In the growth chamber, Bm treatment exhibited PGPR activity and showed increases in shoot and root biomass. Previous work had provided evidence of PGPR traits associated with this strain, including biological nitrogen fixation, inorganic phosphorus solubilization, and IAA production (Brito-Campo et al., 2022). Under these conditions, the PGPR activity of Bm was not affected by co-treatment with HA. This could be explained by three possible factors: 1) the possible PGPR mechanisms of Bm are not necessarily biostimulation; therefore, they do not affect signaling pathways that affect the plant hormonal balance. 2) Bm’s IAA production is low (µg mL-1) compared to other PGPR strains (Brito-Campo et al., 2022); therefore, the HA combination might not represent an additive effect that increases auxin-like activity to a deleterious level to the plant (Pantoja-Guerra et al., 2023). 3) The used plant model (corn) is a monodicotyledonous plant, usually resistant to exogenous auxin activity (McSteen, 2010; Nelissen et al., 2016; Conklin et al., 2019); thus, in the presence of a bacterial strain with low IAA production, higher doses are likely required to express an additive effect that reverses the PGPR activity of the strain.
In field experiments, the shoot biomass results were similar to those obtained under growth chamber conditions. All treatments promoted foliar biomass accumulation. As previously explained, this result is attributed to the hormone-like effects of HA and the PGPR activity of Bm. However, in this test, the HAC treatment did induce increases in shoot biomass. It should be noted that although the concentration of HAs evaluated in the field was the same than that in the growth chamber, the doses (sprayed volumes) were higher in the field. We speculate that an increase in the dose generated a “compensating effect” that allowed the aromatic and carboxyl groups -associated with reactivity in the HAs from coal (García et al., 2016a) to be sufficient to express bioactivity.
The root biomass production results in the field test indicate a PGPR effect of Bm, and it was not affected by the co-application of HAVC. These HAs did not promote the development of roots by themselves, neither under growth chamber nor in field conditions. Supramolecular analysis indicates that these HAs have a lower content of carboxylic and aromatic groups associated with low auxin-like activity (García et al., 2016a; Araújo et al., 2021). This is consistent with the result of the coleoptile elongation test, in which HAVC bioactivity was only observed at the highest concentrations. Therefore, it is reasonable to infer that the combination of Bm with these HAs is not negative for root production in corn, because the "sum of auxin-like effects" is not deleterious under these conditions (Pantoja-Guerra et al., 2023).
Under field conditions, Bm had a positive effect on the number of cobs per plant. However, this effect was reversed by the co-application of HAC and HAVC, thus suggesting that biostimulation caused by both types of HA has a possible additive effect that affects Bm’s PGPR activity. This could be associated with several HA-related hormonal effects.
CONCLUSION
Bioactivity patterns associated with the structural differences of humic acids were observed. HAC presented higher oxygen content, while HAVC presented higher carbon content. On the other hand, the 13C-CP-TOSS-NMR and FTIR analyses show spectral patterns for each sample type, which indicates differences in the composition of functional groups. HAC presented higher aliphatic and aromatic content, while HAVC presented higher aromatics and carboxylic groups content.
Supramolecular composition patterns were correlated with the auxin-like effect of both HA types. The coleoptile elongation test showed differential bioactivity patterns according to the dose. This correlation suggests a possible causal association between HA bioactivity and their supramolecular configuration, as has been reported by other researchers.
Regarding the effect in-planta (growth chamber and field conditions), the HA bioactivity modulated the PGPR effect of the Bacillus mycoides BSC25 strain depending on the sample origin. These results suggest that HA bioactivity should be estimated for the joint application of AH and PGPR strains.