Introduction
Good soil physical characteristics are essential to ensure satisfactory crop and pasture productivity. Pasture soils tend to have greater soil density than preserved vegetation soil, presumably due to trampling by animals (Vitorino 1986), which can also have an impact on the water infiltration rate and soil moisture holding capacity, both of which have significant effects on pasture productivity. The amount of water that infiltrates and flows over the ground is directly related to soil physical properties such as density, and the existing vegetative cover (Lanzanova et al. 2007).
Soil organic matter (SOM) has a major influence on ecosystem productivity because it affects chemical and physical characteristics of soils. Since SOM is the net result of soil processes occurring in the long term, it is difficult to detect early changes if analyzing total SOM (Haggerty and Gorelick 1998). The light fraction of the SOM is formed by plant and animal residues in the early stages of decomposition. It represents recent changes in land management and can detect early changes in SOM dynamics (Jinbo et al. 2007; Rangel and Silva 2007). Increases in ecosystem primary productivity lead to increasing residue deposition, both above- and below-ground.
Silvopastoral systems improve soil physical attributes such as soil aggregates, soil density and water infiltration rates (Carvalho et al. 2004). Litter deposition from tree foliage is a major pathway for recycling of nutrients in a silvopastoral system (Apolinário et al. 2016). Limited nitrogen (N) availability in warm-climate grasslands is one of the major limiting factors to increases in productivity (Vendramini et al. 2014), and N addition via litter represents a significant input and might result in greater ecosystem primary productivity. Tree legumes such as sabiá (Mimosa caesalpiniifolia Benth.) and gliricidia [Gliricidia sepium (Jacq.) Kunth] can be used in silvopastoral systems (Souza and Espíndola 2000; Vieira et al. 2005; Apolinário et al. 2016; Costa et al. 2016). Besides biological N2 fixation, litter deposition and decomposition are important sources of nutrients to be reused by the system (Apolinário et al. 2016).
Tree legumes can provide extra alternative income through the sale of fencing posts and firewood (Apolinário et al. 2015). Incorporating tree legumes in silvopastoral systems can also provide other ecosystem services including the maintenance of biodiversity, improvement of water and nutrient flow, enhancement of soil quality, reduction of soil erosion, improvement of C storage and provision of green areas for urban society (Kemp and Michalk 2005).
Given the economic and environmental importance of these systems, this study aimed to evaluate the chemical composition and physical properties of soils in signalgrass pastures [Brachiaria decumbens Stapf; now: Urochloa decumbens (Stapf) R.D. Webster], in association with tree legumes in the coastal region ("Zona da Mata") of Pernambuco State, Brazil.
Materials and Methods
The research was conducted at the Experimental Station of the Agronomic Institute of Pernambuco (IPA), located in Itambé, Pernambuco, Brazil. Average annual rainfall is 1,300 mm, and average annual temperature is 25 °C (CPRH 2003). The climate is sub-humid, the topography is undulating and the soil of the study area is classified as Ultisol (red-yellow dystrophic Argissol according to the Brazilian Soil Classification or Paleudult or Ferric Luvisol according to FAO World Reference Base) (Jacomine et al. 1972; Embrapa 2006). Initial soil chemical characteristics of the experimental area were: pH in water (1:2.5) 5.5; P (Mehlich-I) 2.2 mg/dm3; K 1.3 mmolc/dm3; Ca 27 mmolc/dm3; Mg 20 mmolc/dm3; Na 1.4 mmolc/dm3; Al 2.7 mmolc/dm3; H+Al 61.7 mmolc/ dm3; and SOM 44.2 g/kg. Average monthly rainfall for the experimental years is shown in Figure 1.
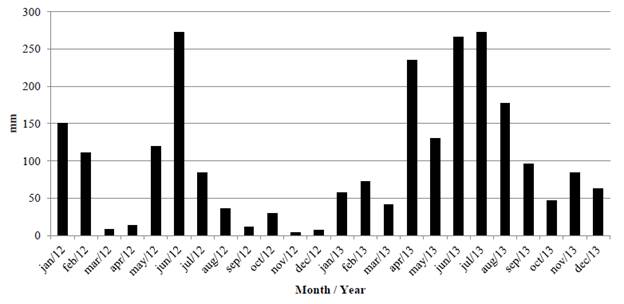
Figure 1 Rainfall (monthly averages) in the experimental area during the research period. Source: Meteorological data collected at the experimental site.
Three treatments were tested in a complete randomized block design with 4 replications. Treatments included: 1) sabiá with signalgrass; 2) gliricidia with signalgrass; and 3) signalgrass monoculture. Each experimental unit measured 660 m2 (33 x 20 m). Tree legumes (sabiá and gliricidia) were established in 2008 in double rows spaced at 10.0 m (between double rows) x 1.0 m (between rows) x 0.5 m (within rows). Each plot contained 3 double rows. The signalgrass was growing throughout the area of each plot, but reduced growth occurred between the individual tree legume rows that formed the double rows ("within tree legume double rows" from here on), especially under sabiá trees. Livestock were introduced to the paddocks when the sward height reached 60 cm, and remained until the stubble height of the grass was reduced to 10-15 cm.
Soils from tree legume paddocks were sampled in September 2012 in order to determine the chemical composition. Samples were collected in 2 transect lines perpendicular to the tree rows. Along each transect, 5 different points were sampled (0, 1, 2, 3 and 4 m distance from each tree double row) giving 30 samples per plot (Figure 2). Paddocks with signalgrass monoculture were sampled randomly at 5 sites. All soil samples were taken from the 0-20 cm soil layer. Soil samples to determine bulk density and soil gravimetric moisture were collected in May 2013, using the same sampling protocol (perpendicular transects) described to collect the soil fertility samples, and the same soil depth. Undisturbed soil cores were collected using volumetric rings. Samples were dried in an oven at 105 °C for 24 hours, following methodology described by Embrapa (1979).
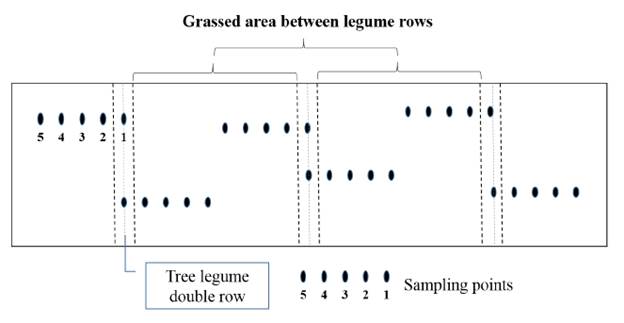
Figure 2 Location of soil sampling points relative to tree legume rows in the silvopasture treatments.
Light fraction of SOM was determined by weighing 50 g of soil (samples collected for fertility analyses), which were macerated and sieved through a 0.5 mm sieve, and then put into a 0.053 mm sieve and washed in running water. The retained material was then transferred to containers filled with water, where it remained undisturbed for 24 h for density separation (heavy and light fraction). The supernatant (floating) material was retrieved in 0.053 mm mesh, dried at 65 °C for 72 h, and weighed on a precision scale (Correia et al. 2015).
Water infiltration rate (WIR) was determined in January 2013. Infiltrometers made of concentric rings (Bouwer 1986) were placed at 2 specific points in each tree legume paddock: 1) within tree legume double rows (sampling point 1); 2) in the middle of the grassed area (sampling point 5). A total of 48 samples were collected (2 replicates for each sample location within each silvopastoral system, and 4 samples for each signal-grass plot). Water infiltration rate was determined when the rate was constant, using the following equation:
WIR (mm/min) = L2 - L1 (mm) / time (min) where: L2 is water at the beginning of the measurement and L1 is the remaining water in the tube after the time spent measuring.
Soil attributes were analyzed using PROC MIXED (SAS 2007). A complete randomized block design was used to compare signalgrass monoculture with the silvopastoral systems. When transects were analyzed, the transect points were considered split-plot and the main plot the vegetation cover, with both being fixed effects. In all analyses, blocks were considered a random effect. Significance was declared at 5% probability. LSMEANS were compared using the PDIFF procedure and adjusted Tukey test.
Results
Soil fertility
While soil chemical composition was affected by vegetation cover (Table 1), levels of most nutrients were similar in all treatments (P>0.05). Soil pH was greater in the signalgrass monoculture than in the 2 grass-legume tree pastures. Soil exchangeable Ca was greater in the grass-legume tree pastures than in the grass-only pasture, and soil exchangeable Na was greater in the signalgrass-sabiá pasture than in the other pastures (Table 1). When com-paring the sampling points in relation to the distance from the rows of legumes, there was no significant effect for the response variables evaluated, except for pH (Figure 3), where values increased exponentially as distance from the legume rows increased, with a peak at 3 m.
Table 1 Soil chemical analyses (0-20 cm layer) in signalgrass, signalgrass-gliricidia and signalgrass-sabiá pastures.

Values followed by the same letter within columns do not differ by Duncan's test (P>0.05). OM = organic matter.
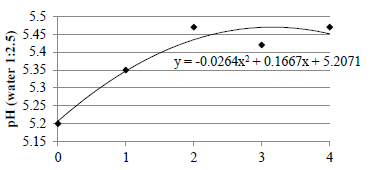
Figure 3 Soil pH relative to the distance from tree legume rows in signalgrass-gliricidia and signalgrass-sabiá pastures.
Water infiltration rate
Water infiltration rate was higher within the tree legume double rows of gliricidia and sabiá (356 and 366 mm/h, respectively; Figure 4) than in the signalgrass monoculture (162 mm/h) and in the grassed area of the signal-grass-sabiá (128 mm/h) treatment.
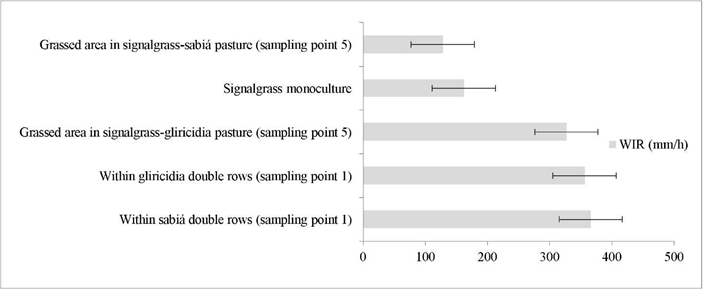
Figure 4 Water infiltration rate (mm/h) in signalgrass monoculture and grassed areas in signalgrass-gliricidia and signalgrass-sabiá pastures, and within gliricidia and sabiá double rows in the mixed pastures. The bars represent the standard error.
Gravimetric moisture
Soil moisture (Table 2) levels were higher (P<0.05) in the signalgrass monoculture than in the mixed pastures; in the mixed pastures soil moisture increased as distance from the tree rows increased (P<0.05; Table 3).
Table 2 Soil moisture and density (0-20 cm layer) in signal-grass monoculture, signalgrass-gliricidia and signalgrass-sabiá pastures.
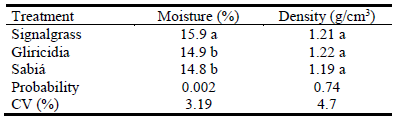
Values followed by the same letter within columns do not differ by Duncan's test (P<0.05).
Table 3 Effect of distance from tree legume rows on soil moisture, soil density and soil organic matter (SOM) light fraction (0-20 cm layer) in signalgrass-gliricidia and signalgrass-sabiá pastures.

Values followed by the same letter within columns do not differ by Duncan's test (P>0.05).
Soil density was not affected by type of pasture (P = 0.58) (Table 2), but in the mixed pastures soil density increased as distance from the tree rows increased (P<0.05; Table 3).
Light fraction of soil organic matter
Light fraction of SOM was unaffected by pasture type (P = 0.22), but within the silvopastoral treatments, light fraction of SOM was greater in the sabiá treatment than under gliricidia (64 vs.45 mg/kg, respectively; P = 0.002) (Table 4). The light fraction of SOM was greater under the trees than in the grass area (71 vs. 50 mg/kg, respectively; Table 3).
Table 4 Soil organic matter (SOM) light fraction (0-20 cm layer) in signalgrass-gliricidia and signalgrass-sabiá pastures.
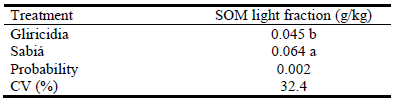
Values followed by the same letter within columns do not differ by Duncan's test (P>0.05).
Discussion
This study has provided some interesting results on changes in soil parameters when tree legumes are introduced into a pure grass pasture. They contribute to our knowledge of how the legumes alter the soils in conjunction with an associated grass.
Soil fertility
As in our study, Carvalho et al. (2003) reported increased soil exchangeable Ca in silvopastoral systems 5 years after establishment, and attributed this increase to the greater accumulation of litter produced by the trees. Similarly, Camarão et al. (1990) suggested that the increase in soil exchangeable Ca in silvopastoral systems might be explained by the increased above- and below-ground litter deposition. Xavier et al. (2003) also observed an increase in soil exchangeable Ca in signalgrass-Acacia mangium pasture compared with pure signalgrass.
The reduction in soil pH in the mixed pastures recorded in our study is in contrast with the findings of Oliveira et al. (2000), Andrade et al. (2002), Xavier et al. (2003) and Dias et al. (2006), where soil pH was not affected by the introduction of trees. Dias et al. (2006) also studied soil chemical composition of grass-tree legume pastures in relation to the distance from the tree trunk and found variations in soil pH and levels of P, K, Ca and Mg tending to increase or decrease, depending on the legume species, planting density and biomass production.
In silvopastoral systems, most litter deposition occurs near the tree trunks (Silva et al. 2013), which might influence the reduction of soil pH. Greater litter accumulation leads to greater amounts of litter nutrients being mineralized. As a result, more leaching of exchangeable bases due to release of anions from OM might occur (Balbinot et al. 2010). However, Pavan et al. (1986) obtained an increase in soil pH in an area with greater litter deposition. Several studies on silvopastoral systems indicated that the benefits brought by the trees to soil fertility of the pastures tend to increase over time with development of the trees, increase in litter deposition and accumulation of animal waste, provided that the system is appropriately managed (Balbino et al. 2012; Padovan and Pereira 2012; Loss et al. 2014).
Water infiltration rate
Greater WIR in the signalgrass area in consortium with gliricidia might be due to the fact that this legume has a deeper root system, providing advantages such as increased water absorption and greater efficiency in the search for nutrients, resulting in its high performance as fodder for livestock (Abdulrazak et al. 1997; Ondiek et al. 1999; Juma et al. 2006). A more specific study of the root systems of gliricidia and sabiá is necessary to better understand the influence of root properties (length, depth and density) on WIR.
Silvopastoral systems allow increases in SOM because of greater litter deposition from trees, and Bell et al. (2011) indicated that greater litter deposition increases soil macroporosity, contributing to improved water infiltration and aeration. Moisture, biological activity and vegetation cover can also influence soil responses, such as the WIR (Carduro and Dorfman 1988). Dunger et al. (2005) reported that silvopastoral systems provide a favorable microclimate to increase soil microfauna, which tend to seek shaded and humid habitats. An increase of Coleoptera beetles in association with the introduction of legumes from the genus Mimosa in pastures has been reported by Dias et al. (2007). These beetles dig underground galleries in order to nest, thus providing the opportunity for greater water infiltration (Miranda et al. 1998).
Increased height and density of tree legumes in the experimental area reduced the transit of grazing cattle through the rows, which might explain the lower soil density at these points (Table 3). The WIR was greater along tree legume rows as compared with the grazed area under the effects of treading by animals, as indicated with changes in soil density. These data corroborate those of Lanzanova et al. (2007), who studied the effects of grazing on water infiltration rates in soils, finding greater WIR values in ungrazed areas and decreasing values as grazing became more intense. In our research, the increases in soil density as distance from tree legumes increased (Table 3) was reflected in decreases in WIR. Bertol et al. (2001) showed that heavy clay soils have a low percentage of the pore volume occupied by air, which leads to greater rates of runoff water, lower retention of water and consequently lower infiltration capacity. Prevedello (1996) also pointed out that the reduction in WIR with time can be influenced by factors that operate on the soil surface, such as surface sealing due to the impact of raindrops, which may be reduced by the canopy of tree legumes. Roots of tree legumes in the silvopastoral systems used in this experiment might favor soil physical aspects, maintaining and improving soil structure and increasing WIR (Hernández 1998).
Excretion of organic acids and inorganic compounds (e.g. P and K) by roots can influence soil characteristics, as they allow for increased dissolution of mineral substances and contribute to the development of rhizosphere microorganisms (Cintra et al. 1999). Roots can also favor SOM accumulation, as Lehmann and Zech (1998) found that the litter produced by the renewal of roots adds about 20-50% of the total root biomass to the SOM pool, while only 10-20% of litter arising from the aerial parts is transformed into SOM (Schroth et al. 1999). Since roots are more recalcitrant than leaves and stems, a greater proportion of original root biomass ends up in the SOM pool than leaves and stems.
Gravimetric moisture
The greater soil moisture in signalgrass monoculture was probably due to the competition by different species for water. Legumes are less efficient in water usage than C4 grasses. On average, legumes use 800 kg of water to produce 1 kg of dry matter, while C4 plants use 300 kg of water to produce the same amount of DM (Taiz and Zeiger 2004; Marenco and Lopes 2009). Plant species have a marked influence on water availability in silvopastoral systems and Vanzela and Santos (2013) highlighted that the use of eucalypts in silvopastoral systems increased competition for water and nutrients between the trees and the associated grass.
Andrade and Valentim (1999) showed that shading is a positive factor in maintaining soil moisture, resulting in satisfactory forage development in silvopastoral systems. In natural shading conditions, however, trees also compete with one another and the grass for light, water and nutrients. Therefore, the water requirements of the tree legumes might have contributed to reduced soil moisture near the trees in the current research.
Another aspect that should be highlighted is the fact that, during the collection period, the grass monoculture was approximately 60 cm tall, which provided 100% ground cover, helping to maintain soil moisture. In the silvopastoral systems, tall trees with dense canopies might have compromised production of signalgrass, which has only moderate shade tolerance and might suffer production loss due to shading (Schreiner 1987). In contrast to this, Aguiar et al. (2006) recorded greater soil moisture in silvopastoral systems compared with agrosilvopasture (combination of trees, crops and livestock, grown on a particular site) and intensive cultivation. Perin et al. (2000) also observed greater soil moisture when soil was covered with a thick litter layer of herbaceous legumes.
The increase in soil moisture as distance from the tree legume rows increased meant that grasses growing in the middle of the grass strips suffered reduced competition for soil moisture from the trees, while still having some shade to assist retention of soil moisture (Table 3). Near tree rows, there was reduced soil cover because of greater competition for resources between herbaceous and woody vegetation.
Soil density
Average soil density was 1.2 g/cm3, which is adequate for root development (Alvarenga et al. 1996; Corsini and Ferraudo 1999). According to Argenton et al. (2005), characterization of soil density depends on its textural class and Rosenberg (1964) and Cintra and Mielniczuk (1983) suggest that each soil type has a critical density, which can reduce or even prevent root development. Reichert et al. (2003) showed that 1.4 g/cm3 is considered the critical soil density for satisfactory growth of the root system of plants in clay soils, but Reinert et al. (2008) indicated a greater soil density (1.85 g/cm3) as critical for legumes and other vegetables in clayey soils.
The lower soil density near the trees (Table 3) can be attributed to the existence of microfauna near the trees (Miranda et al. 1998; Dunger et al. 2005; Dias et al. 2007) as well as a greater SOM accumulation between trees, increasing the amount of soil aggregates. Iori et al. (2012) studied soil density and soil moisture in degraded pastures, banana cultivation, a silvopastoral system and preserved forest. They found greater soil moisture in less dense soil, which can be correlated with the shading potential and greater SOM in these areas. Beltrame et al. (1981) stated that soil moisture affects the cohesion between soil particles, with increases in aggregation when soil moisture is limited, which hinders their separation by external forces (Silveira et al. 2010).
Light fraction of soil organic matter
While vegetation cover did not affect the light fraction of SOM (P = 0.22), in the mixed pastures sabiá presented greater values of SOM than gliricidia (Table 4). Chan et al. (2002) and Zinn et al. (2005) observed that SOM stocks are directly related to residue inputs, their rate of decomposition and SOM fractionation. They pointed out that the replacement of conventional farming systems with improved systems, such as silvopastures, changes the dynamics of litter accumulation and litter decomposition rate, and consequently generates greater increases in the light fraction of SOM. Similarly, Maia et al. (2008) showed greater amounts of light fraction of SOM in silvopastoral systems (38.2 g/dm3) than in conventional tillage (28.4 g/dm3), because of greater litter input from trees. The amount of light fraction in the system is directly related to the litter deposited on the soil. Light fraction of SOM is composed of litter and organic matter in intermediate stages of decomposition (Souza et al. 2006) and its level at any given time is the net balance between its deposition and decomposition (Fraga 2002).
Phenolic substances found in plants often influence litter decomposition rate and, consequently, nutrient cycling, affecting the composition and activity of decomposing communities of the system (Háttenschwiler and Vitousek 2000). Among these substances, flavonoids are characterized by their recalcitrance, with condensed tannin (CT) concentration usually correlating with low decomposition rates (Burhenne et al. 2013). Nozella (2001) found high levels of condensed tannins (near 6.9 g/kg DM in gliricidia), while Balogun et al. (1998) determined mean values of 0.8%. Beelen (2002), however, showed greater values in sabiá, reaching up to 20.1%. Greater CT concentration in sabiá might explain the greater light fraction of SOM observed in the silvopasture with this species, compared with the one with gliricidia.
Conclusions
This study has shown that incorporation of tree legumes in rows within a signalgrass pasture can improve soil chemical composition over time as well as increasing WIR in the soil, and the concentration of light fraction SOM near the trees. These findings indicate that silvopastoral systems using tree legumes can potentially serve as greater C sinks than pure grass pastures as well as providing other services to farmers. However, long-term results coupled with life cycle assessments are necessary to determine what productivity increases will result.