More than 90% of environmental pollutants, such as heavy metals, are retained in soil particles. Lead contamination is significant because this heavy metal has a long residence time, persisting for 1000-3000 years (Rodríguez et al. 2016). Environmental exposure to lead (1,300-32,260 mg kg-1 in soil) affects public health worldwide (Covarrubias and Peña 2017) and is the cause of 143,000 deaths per year (Rodríguez et al. 2016). Lead (0.13-446 ppm) has been quantified in irrigation water contaminated with industrial residues or remains of fertilizers, in vegetables eaten fresh, strawberries, potatoes, cassava, sugar cane juice, and industrial fruit juices and vegetables (Salas-Marcial et al. 2019), a condition that constitutes a potential problem in food safety for humans and animals.
Lead enters the body through the respiratory, digestive, and dermal routes (Covarrubias and Peña 2017). Lead is not essential for plants and does not have any biological function, but it is transported to plant tissues. Once inside, the heavy metal increases the production of reactive oxygen species (ROS) that affect lipid cell membranes and reduce photosynthetic activity, with symptoms such as chlorosis, necrosis, decreased root and foliage growth, as well as changing root branching patterns (Nakbanpote et al. 2016).
Resistant plants present mechanisms such as evasion and tolerance that allow them to survive with high concentrations of heavy metals. In evasion, root cells limit and restrict the uptake and movement of heavy metals in plant tissues through the mechanisms of root uptake, metal precipitation, and exclusion. Exclusion barriers between roots and foliage minimize the accessibility of metals present in the soil to the roots (Sabreena et al. 2022). When the mechanisms are not adequate to reduce toxicity in plants, ROS are formed in the cytoplasm, which causes oxidative stress. In this context, plants activate enzymatic and non-enzymatic antioxidant defense mechanisms to overcome the negative effect of ROS (Sabreena et al. 2022).
Phytoremediation is defined as the use of plants and associated microorganisms to reduce contaminants in water, soil, and air. Also, It does not contaminate the environment and can be used to extract or immobilize metals, metalloids, and radionucleotides, as well as organic xenobiotics (Rigoletto et al. 2020; Sabreena et al. 2022). However, in some cases, the remaining concentrations exceed the maximum permissible limits established by the regulations to consider it as bioremediated soil (MINAM 2017). Phytoremediation can be improved with strategies that favor and accelerate the process, such as the synergy between plants and plant growth-promoting rhizobacteria (PGPR). These plant-associated microorganisms modify the bioavailability of lead through the production of siderophores, organic acids, exopolysaccharides, and solubilization of precipitated phosphates (Shah and Daverey 2020).
Weeds are fast-growing and can be used for phytoremediation of soils contaminated with lead (Contreras-Pinto et al. 2016). However, before its use, it is necessary to investigate its capacity to reduce the contaminant alone, and in combination with rhizosphere microorganisms, with the perspective of overcoming the limitations that affect the process. For this reason, this study aimed to determine the potential of weeds and associated rhizobacteria for soil phytoremediation of a solid waste dump contaminated with lead.
MATERIAL AND METHODS
Sampling location
The municipal solid waste dump, located in Lambayeque, Peru, covers an area of 2.95 km2 between the parallels 06° 55' 04" South latitude and 79° 44' 07" West longitude. An identification sampling (IS) was carried out, aimed at determining if the soil was contaminated in an area of 1 ha (0-10 cm depth). The sampling pattern was of random distribution with regular grids, for which nine grids or cells (333.5 m2) were delimited with parallel and perpendicular lines (MINAM 2017). In 1 m2 squares delimited in the central part of each cell, the 2 cm of surface soil was removed. In each square, 24 kg of soil (10 cm deep) were collected and deposited in polyethylene bags.
The soil from the nine sacks was mixed on a blanket and homogenized using the conning and quartering method (Contreras and Carreño 2018), and two representative samples (1 and 2 kg) were collected. In the 2 kg sample, the physical and chemical analysis was performed, while in the 1 kg sample, the count of total microorganisms was performed using the most probable number (MPN) technique in nutrient broth and the MPN of lead-tolerant microorganisms in nutrient broth with 50 mg of Pb L-1. The rest of the soil was kept in the greenhouse of the Pedro Ruiz Gallo National University for the experimental phase of this research. The soil had a sandy texture, non-saline condition (EC=0.41 dS m-1), organic matter (0.02%), nitrogen (0.006%), phosphorus (3.7 ppm), potassium (131 ppm), lead (11.91 mg kg-1), cadmium (1.12 mg kg-1) and chromium (16.94 mg kg-1).
Selection of lead-tolerant weeds
In six agricultural fields, six specimens of 15 weeds with similar height and at the beginning of flowering were selected. With a shovel, the plants with their roots and rhizospheric soil were extracted and then transplanted into polypropylene pots (4 kg capacity) with 3 kg of soil from the municipal waste dump, mixed with 0.3 kg of rice husks.
Two specimens of Echinochloa colona (L.) Link, Cyperus corymbosus Rottb. and Sorghum halepense were planted in the pots (three pots per weed) and irrigated with drinking water (stored for 24 h) twice a week. Weeds were classified as adapted to transplanting if their vigor and green color were maintained and they developed leaves or shoots the following 10 days. In the adapted weeds, the tolerance to lead was investigated, for which four of the six plants were irrigated with a solution of lead nitrate Pb (NO3)2 at a concentration of 10 ppm of Pb once for the first, second, and third weeks. Since the fourth week, irrigation was carried out every 3 days with a Pb (NO3)2 solution, whose concentration increased geometrically for five weeks: 50, 100, 200, 400, and 800 ppm of Pb. The two remaining specimens of each weed were irrigated with lead-free water and constituted the reference controls of the normal phenotype.
Lead tolerance was qualified by the survival of 100% of the plants, and the physical appearance similar to the controls, in terms of height, vigor, and color of the leaves. After 60 days of the first irrigation with the Pb (NO3)2 solution, the biomass of the plants was weighed and the stress tolerance index was calculated according to Chaturvedi et al. (2020) method. Weeds tolerant to Pb (800 ppm) were those that presented tolerance indices of 0.5-0.8, while highly tolerant weeds presented indices greater than 0.8 according to Frachia et al. (2022).
Plant growth promotion by rhizospheric bacteria
The rhizospheric soil (10 g) of lead-tolerant weeds was inoculated into 100 mL of nutrient broth and incubated at 30 °C for 5 days (Gupta et al. 2018). The bacteria were isolated using nutrient agar supplemented with 50 mg L-1 of Pb, incubated at 30 °C for 5 days. The developed colonies were grouped according to their morphological characteristics (morphotypes) and reaction to Gram staining. A representative of each morphotype was selected, and those that grew (turbidity) in nutrient broth with 100, 200, 400, and 800 mg L-1 of Pb were cultured in nutrient agar with and without lead, and incubated at 30 °C for 48 h, constituting the pure cultures of lead-tolerant bacteria (Manzoor et al. 2019).
The characteristics that show the in vitro plant growth promotion was investigated in lead-tolerant bacteria. The phosphate-solubilizing activity of bacteria was preliminarily evaluated on Pikovskaya agar by observing the appearance of a translucent halo around the colonies after 96 h of incubation, while the quantification of phosphate solubilization was evaluated in Pikovskaya broth. On the other hand, indole acetic acid (IAA) production was evaluated in Tryptic Soy Broth (TSB) plus the Salkowski reagent, according to Liu et al. (2022).
The soluble phosphorus concentration was calculated with equation 1 using a wavelength of 690 nm, and the IAA concentration with equation 2 using a wavelength of 530 nm.
Where X1 is the soluble phosphorus concentration (ppm), Y1 is the corrected absorbance of bacterial sample ‘n' minus the absorbance of the culture medium control (0.119); X2 is the concentration of IAA (ppm), Y2 is the corrected absorbance of bacterial sample ‘n' minus the absorbance of the culture medium control (0.080).
Influence of rhizospheric bacterial consortia on the aboveground biomass of weeds and phytotoxicity of the phytoremediated soil
The soil (50 kg) previously collected in the dump was sieved (<5 mm), mixed with 5 kg of rice husk, autoclaved at 121 °C for 20 min (Chaturvedi et al. 2020) and conditioned in 18 pots, at a rate of 3 kg per pot. Next, the soil of nine pots was artificially contaminated with a Pb(NO3)2 solution until reaching 0.127% w/w, equivalent to 800 mg kg-1 of Pb, considered the minimum concentration for commercial/industrial/extractive soil according to the Environmental Quality Standards (EQS) established by MINAM (2017).
After one month, the three lead-tolerant plant species were transplanted in triplicate into pots with lead-contaminated and non-lead-contaminated soil (two plants per repetition). Before transplanting, in the treatments where the bacterial consortia (BC) were inoculated, the roots of plants with a similar phenotype were immersed in a suspension of the corresponding bacterial consortium for 30 min.
The inoculum of the bacteria was obtained by the large-scale sowing method with two volumes: mother and definitive. The bacteria were grown independently in nutrient broth with 800 ppm of Pb. The mother culture was obtained after inoculating 1.8 mL of cultured broth with each bacterium in 16.2 mL of nutrient broth with 800 ppm of Pb and incubated at 30 °C for 24 h. The definitive culture was obtained after inoculating the mother culture (18 mL) of each bacterium in 162 mL of nutrient broth with 800 ppm of Pb and incubated at 30 °C for 24 h. In this way, 180 mL of the definitive culture of each bacterium were obtained, while for the consortia, the definitive cultures of six bacteria per weed were mixed with a total of 1,080 L (Acosta and Bustamante 2020). Finally, at 90 days after transplanting, the fresh aerial biomass of each plant was weighed.
Phytotoxicity of the phytoremediated soil
The phytotoxicity of the phytoremediated soil was determined in seeds of Raphanus sativus L. "rabanito" var. Crimson Giant. In Petri dishes, 10 g of soil from each repetition of the six investigated treatments were deposited in triplicate, moistened with sterilized distilled water, and 25 seeds (in 18 Petri dishes) were conditioned in each plate. Three Petri dishes with uncontaminated soil were included as control. After 120 h at room temperature (20-22 °C), the germinated seeds were selected and quantified, the length of each radicle was measured, and the relative germination percentages (RGP), relative radicle growth (RRG) and the germination index (GI) were calculated according to Lakhal et al. (2017).
The phytotoxicity level was determined by relating the GI to the presence of toxic substances: GI≥ 80%, a very low number of toxic substances with a low level of toxicity, 80%>GI>50%, a moderate number of toxic substances with a moderate level of toxicity, and GI≤50%, an abundant number of toxic substances with a severe level of phytotoxicity. The soil and weeds, both with and without bacterial consortia, which exhibited the lowest levels of toxicity in radish seeds, were collected to quantify lead and determine phytoremediation parameters.
Parameters of lead phytoremediation in soil with the lower phytotoxicity
The roots and foliage of the selected weeds were washed, and together with the soil that accompanied them, were dehydrated in an oven at 75 °C for 96 h (Lu et al. 2021). Both were conditioned and sent to the Soil, Plant, Water, and Fertilizer Analysis Laboratory of the National Agrarian University - La Molina to quantify lead by atomic absorption spectrophotometry (Perkin Elmer, Model PIN 500). The quantified lead values in the soil, roots and foliage were used to calculate the bioaccumulation factor (BAC), the translocation factor (TF) and the percentage of lead removal from the soil (Chaturvedi et al. 2020).
Identification of Phytoremediating Bacteria
The identification of the genus of bacteria in the consortium was carried out by several biochemical like catalase, oxidase, motility, production of acidity from glucose on triple sugar iron (TSI) agar, reduction of nitrates to nitrites, hydrolysis of urea and starch, indole production, citrate utilization, lysine decarboxylation on lysine iron agar (LIA) and gelatin and esculin hydrolysis (Silva et al. 1999; Salazar and Nieves 2005; Álvarez-López et al. 2014; Dipak and Sinha 2017).
Statistical analysis
The data analyzed were represented with the mean ± standard deviation (SD) of three repetitions. Statistical analysis of fresh biomass was performed using variance analysis (ANOVA), followed by Tukey's test (P<0.05). For all the analyses, the statistical program SPSS V. 22.0 was used.
RESULTS AND DISCUSSION
Selection of lead-tolerant weeds
A 20% of the selected weeds were considered tolerant to lead (800 ppm), with tolerance indices of 0.80 (Echinochloa colona (L.) Link), 0.76 (Cyperus corymbosus Rottb.) and 0.72 (Sorghum halepense). The tolerance of weeds to lead (800 ppm) coincides with the reports of Amadi and Gbosidom (2022) with E. colona (L.) Link, and Sabreena et al. (2022) with Sorghum spp. Also, lead decreased the fresh biomass of weeds (Figure 1), a negative effect previously reported by Chaturvedi et al. (2020). The researchers demonstrated that lead (50-100 ppm) decreased plant height (15.38%) and biomass (13.7%), as well as chlorophyll, carotenoids, and nitrogen. On the other hand, Frachia et al. (2022) reported that lead (100-500 ppm) increased the weight of foliage and roots of Inga uruguensis, and based on the fresh biomass of the plants, a tolerance index (TI) of 1.21 was reached.
The morphological and physiological changes of plants in lead-contaminated soils vary according to the concentration of the heavy metal and the plant species (Lu et al. 2021). When the concentration of the contaminant is <500 ppm, an increase in growth parameters has been reported (Liu et al. 2018; Frachia et al. 2022), but a decrease in height and biomass in plants developed with 50-100 ppm Pb was also observed (Chaturvedi et al. 2020).
Plant growth promotion by rhizospheric bacteria
In the rhizosphere of three lead-tolerant weeds, 26 colonies of Gram-negative (76.9%) and Gram-positive (23.1%) bacteria were isolated on nutrient agar with 50 mg L-1 of Pb, among which 92.3% grew in nutrient broth with 100 and 200 mg L-1 of Pb, 76.9% in broth with 400 mg L-1 of Pb, and 50% in broth with 800 mg L-1 of Pb. In the rhizospheric soil of E. colona (L.) Link, 10 cultures of bacteria were obtained, and 60% of Gram-negative bacteria grew with 800 mg L-1 of Pb. In the rhizospheric soil of C. corymbosus Rottb., seven cultures of bacteria were obtained that grew with 400 mg L-1 of Pb, and 71.4% with 800 mg L-1 of Pb. In the rhizospheric soil of S. halepense, nine cultures of bacteria were obtained, among which 44.4% grew in broth with 400 mg L-1 of Pb, and 22.2% in broth with 800 mg L-1 of Pb.
Similar results were reported by Gorelova et al. (2022) and Manzoor et al. (2019), who isolated microorganisms in the rhizosphere of plants in soil contaminated with lead artificially and by anthropic activity, respectively. Manzoor et al. (2019) determined 62% of lead tolerant Gram-negative bacteria. Moreover, Liu et al. (2018) determined significant changes in the microbial population developed in a lead-contaminated site, an increase in the relative abundance of the bacterial genera Flavisolibacter, Kaistobacter, and Pseudomonas, as well as a decrease in the genera Bacillus, Adhaeribacter, Pantibacter, and Paenibacillus. The rhizosphere is the soil close to the roots (5 mm) that is directly influenced by root exudates, and where the largest population of microorganisms that interact with plants is found (Gamalero and Glick 2022).
All the microorganisms from bacterial consortium one (BC1) solubilized tricalcium phosphate and produced AIA (Table 1), characteristics that show in vitro growth promotion in plants. Phosphate solubilizing bacteria produce organic acids that destabilize the precipitated mineral and release soluble phosphorus to plants (Gavrilescu 2022). The increase in phosphorus availability favors plant growth and metabolite processes such as photosynthesis, energy transfer, and carbohydrate utilization (Corrales et al. 2017). Likewise, rhizospheric microorganisms produce auxins such as IAA that induce the proliferation of the root system, cytokinins related to cell growth and regulation of hormones such as ethylene through the activity of the enzyme amino 1-carboxylate (ACC) deaminase, in addition to nitrogen fixation, phosphate solubilization, siderophore synthesis and ammonium production (Gavrilescu 2022).
Influence of rhizospheric bacterial consortia on weed growth and phytotoxicity of phytoremediated soil
The consortia of rhizospheric bacteria positively influenced the growth of lead-tolerant weeds, observing increases of 14.32% (E. colona (L.) Link + BC1), 4.60% (Cyperus corymbosus Rottb. + BC2) and 4.14% (Sorghum halepense + BC3). Tukey's mean comparison test showed that the highest value of fresh biomass corresponded to the E. colonum plus BC1, with significant differences compared to the other treatments (Figure 2), a similar result to the reported by Manzoor et al. (2019) in the ornamental plants Pelargonium hortorum and Mesembryanthemun criniflorum grown in soil artificially contaminated with 500-2,000 mg kg-1 of Pb. The researchers showed that Bacillus tequilensis increased the biomass of both plants in soils with and without lead concentrations. There was an increase of 65.52% in M. criniflorum and 64.04% in P. hortorum in the soil with 2,000 mg kg-1 of Pb.
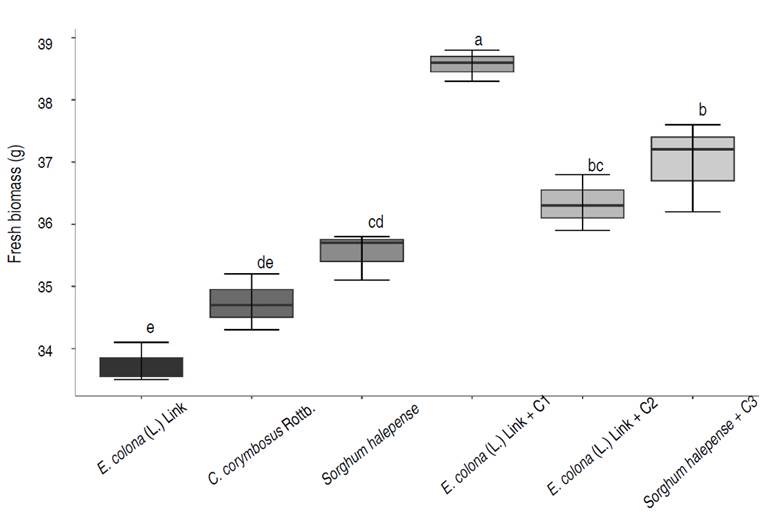
Figure 2 Fresh biomass of weeds at 90 days after inoculation of lead-tolerant bacterial consortia (BC). Different lowercase letters indicate significant differences between treatments (P<0.05).
Liu et al. (2018) showed that 100 ppm of lead did not affect the growth of T. repens; however, 500 ppm of lead significantly decreased root length (19.08%), plant height (25.92%), aerial biomass (25.1%) and root biomass (24.3%).
The level of phytotoxicity was from severe to moderate in the soil where the lead-tolerant weeds were grown for 90 days, and it was from moderate to low in the soil with the lead-tolerant weeds inoculated with the bacterial consortia. The soil where the highest germination rate of radish seeds was reached (89.56%), and therefore the lowest level of phytotoxicity (low) was that corresponding to E. colona (L.) Link with BC1 (Table 2).
Parameters of lead phytoremediation in soil with the lower phytotoxicity
Lead phytoremediation parameters in soil with lower phytotoxicity, planted with E. colona (L.) Link increased with the inoculation of bacterial consortia. In the E. colona (L.) Link inoculated with BC1, the bioaccumulation factor (BAC) was 0.1651 in the foliage, and 1.0250 in the roots; the translocation factor (TF) was 0.1611 compared to 0.0115, 1.0116 and 0.0114 in plants not inoculated with bacteria. In this context, the percentage of lead removal in the soil was 78.83% with E. colona (L.) Link inoculated with BC1, and 57.58% with uninoculated E. colona (L.) Link (Table 3).
Table 3 Parameters of phytoremediation of soil contaminated with lead by Echinochloa colona (L.) Link with and without bacterial consortium 1 (BC1).
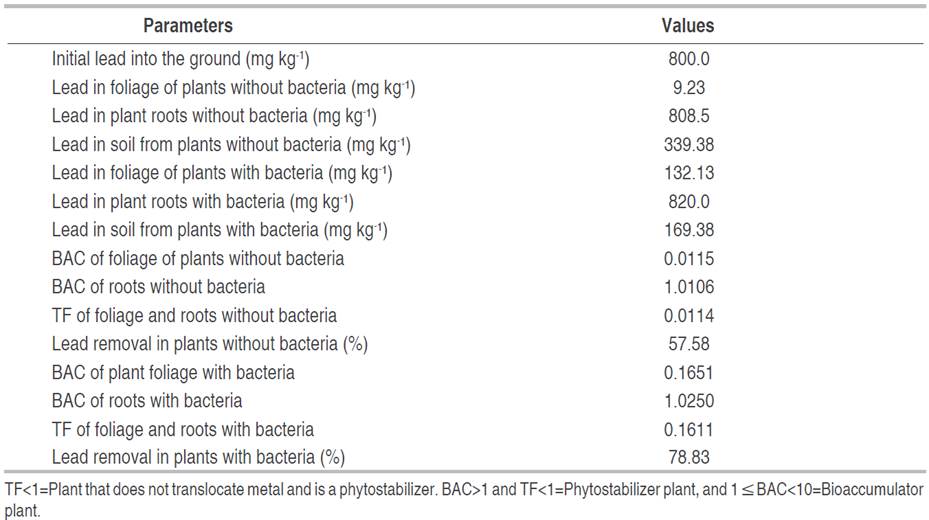
The concentration of lead accumulated in the roots was higher than the foliage of the E. colona (L.) Link plants with and without bacteria, a superiority that coincides with the studies of Gorelova et al. (2022) and Lu et al. (2021). In this regard, Gorelova et al. (2022) quantified 1.57-7.38 mg kg-1 of Pb in roots, and 0.14-0.88 mg kg-1 of Pb in foliage of Echinochloa frumentacea, and concluded that this species is not suitable for remediation, because it accumulates and removes only a small amount of lead (0.18-0.94 g ha-1), compared to other plants such as Sorghum halepense with 107-378 g ha-1. After lead enters the soil, it remains mostly in divalent forms (oxides and sulfides). Precipitated forms of lead phosphate [Pb3(PO4)2] and lead carbonate (PbCO3) are found in the root, which are difficult to transport to the aerial part (Lu et al. 2021). Plants respond to lead stress in different ways, but higher BAC is common in roots compared to foliage (Lu et al. 2021). These researchers determined a BAC range of 0.931-1.062 in the root of Plantago asiatica L. and 0.074-0.087 in the leaves, a result that demonstrated that the root is the main organ for absorption and accumulation of lead.
The bioaccumulation factor (BAC) of lead in the roots of Echinochloa colona (L.) Link with and without bacteria was greater than one and the translocation factor (TF) was less than one, a result that demonstrated this plant species is a phytostabilizer of lead, due to plants with a BF>1 and TF<1 are appropriate for phytostabilization (Rigoletto et al. 2020). The bioaccumulation or bioconcentration factor estimates the absorption capacity of metals in plants, and the translocation factor evaluates the transport of metal from the root to the foliage (Rigoletto et al. 2020). The TF values of 0.1611 and 0.0114 in E. colona (L.) Link with and without bacteria were lower than the range of 0.061-0.819 reported by Lu et al. (2021) in Taraxacum mongolicum and Plantago asiatica L. developed with 5% of lead. According to Rigoletto et al. (2020), plants with a translocation factor less than one are not capable of transporting the contaminant from the roots to the foliage and are used for the phytostabilization of the contaminant. In this way, they immobilize lead and avoid the contamination of groundwater.
The lead content decreased in the phytoremediated soil with and without bacteria, a decrease previously reported by Lu et al. (2021) and Gorelova et al. (2022). At the end of the evaluation period of this study, 57.58% removal of the contaminant was reached in the soil of the plants where bacteria were not applied, and 78.83% when bacteria were applied. The range of percent lead removal is greater than the 13.01-31.89% reported by Lu et al. (2021) in soils contaminated with 2-5% lead and remediated with Artemisia capillaris and Plantago asiatica L.
Microorganisms favor the phytoremediation of contaminated soil directly by reducing the toxic effect and increasing the metal absorbed in the roots and foliage (Manzoor et al. 2019), and indirectly through the promotion of plant growth (Gavrilescu 2022). Growth-promoting microorganisms increase the solubility of lead because they decrease the pH (Manzoor et al. 2019), adsorb lead on their surface and precipitate (Zhu et al. 2022), capture lead by the biosorption mechanism, modify the bioavailability of lead through the production of siderophores, phosphate solubilization, production of organic acids and exopolysaccharides (Shah and Daverey 2020), and transform lead into inert forms such as lead sulfate (PbSO4) and lead sulfides (PbS) (Rigoletto et al. 2020).
Due to the formation of insoluble precipitates, the availability of lead is very low in the soil. In this context, citric and malic acid are used to improve the accessibility of the heavy metal in the soil and the translocation from the root to the foliage (Rigoletto et al. 2020). Therefore, phosphate-solubilizing bacteria of the genera Pseudomonas and Acinetobacter favored the availability of lead, as well as greater absorption by the root system of lead-tolerant plants (Gupta et al. 2018).
Identification of the genus of bacteria in the consortium with the greatest influence on phytoremediation
The genera Pseudomonas and Acinetobacter were identified among the bacteria in consortium one (Table 4). In this regard, it coincides with the microorganisms recovered by Manzoor et al. (2019) (Pseudomonas) and Zhu et al. (2022) (Acinetobacter). The bacteria reported as tolerant to lead, with potential for phytoremediation of soil contaminated with heavy metal, belong to the genera Klebsiella, Pseudomonas, Sporosarcina, Microbacterium, Staphylococcus, Bacillus (Manzoor et al. 2019), Flavisolibacter, Kaistobacter (Liu et al. 2018), as well as the species Acinetobacter calcoaceticus (Zhu et al. 2022), Delftia acidovorans and Azonexus caeni (Abdelrahman and Younggy 2022).
CONCLUSION
The weeds Echinochloa colona (L.) Link, Cyperus corymbosus Rottb. and Sorghum halepense with associated rhizobacteria were efficient in the remediation of soil contaminated with lead. 78.83% of heavy metal removal was achieved with E. colona (L.) Link plus Pseudomonas spp. and Acinetobacter spp. (BC1), compared to 57.80% with E. colona (L.) Link without bacteria. Pseudomonas spp. and Acinetobacter spp. were favored by the root exudates of lead-tolerant plants, which are used as nutrients. This association between weeds and plant growth-promoting bacteria can be applied as an alternative to achieve environmental sustainability through the remediation of soil impacted by several heavy metals.