INTRODUCTION
The genus Prunus belongs to the family Rosaceae in Angiosperms (flowering plants) (WFO, 2024). Peaches (Prunus persica [L.] Batsch) are considered a fruit with a pleasant flavor and aroma. They have many positive properties: anticancer, antiallergic, antitumor, antibacterial, antimicrobial, and anti-inflammatory (Santos et al., 2013; Kant et al., 2018). Additionally, this fruit contains ascorbic acid (vitamin C), carotenoids, phenols, and numerous antioxidants (Gil et al., 2002). The crop is representative of the high-altitude tropical climate of Colombia. In those regions, varieties of peach trees adapted to the tropics are grown (Miranda and Carranza, 2013); and in Colombia during the year 2022, the crop totaled 2,265 ha with a production of 31,307 t (Agronet, 2023). The main producing departments are Boyaca (813.69 ha), Norte de Santander (852.80 ha), Santander (377.50 ha), and Huila (138.50 ha) (Agronet, 2023). In Colombia, there are comparative advantages for production, since by using cold compensators or growth regulators together with cultural practices, up to three harvests per year can be harvested (Castro and Puentes, 2012; Pinzón et al., 2014). This is a comparative advantage over countries in the temperate zones. Unfortunately, one of the main limitations in the production of this fruit are the diseases that limit growth: fungi, bacteria, virus, et al., that cause great losses during harvest and post-harvest, especially in the Department of Boyaca, where varieties such as Golden, Black King, Diamond, and Rubidoux are very susceptible to disease (Guarín Torres et al., 2019).
Besides Colombia, the fungus brown rot (Monilinia fructicola) is very limiting in the producing areas of Australia, Brazil, China, Europe, and New Zealand (Hu et al., 2011). The fungus affects branches, flowers, and fruits, drastically reducing the production and quality of the crops. The M. fructicola cycle is recurrent in the crop causing affected plants to develop blossom rot and cankers on the branches and stems; while in the fruit, it causes rot and mummification (Agrios, 2005).
It is estimated that M. fructicola can cause losses of 50 to 70%, especially in the flowering and fruiting stages that coincide with periods of high humidity and low temperatures. This pathogen also affects loquat crops throughout the world as well as other stone fruit species such as peach, plum, cherry, apricot, and pome fruits such as pear and apple (Agrios, 2005; Michailides et al. 2007; Luo, 2017; Yin et al., 2021).
Monilia fructicola is considered an EPPO A2 pest recommended for regulation since 2004 (it was an A1 pest since 1984), and it is also an A1 quarantine pest according to the Inter-African Phytosanitary Council (IAPSC) since 1989 (EPPO, 2022). Currently, Colombia exports peaches to Swiss, Aruba, and Curaçao (OEC, 2024), so due to the relevance of the disease on fruit of export quality, control is obligatory in the country. Control of the disease is carried out through the application of chemical fungicides and other control practices such as pruning and thinning of diseased flowers, twigs, and fruits. But the continuous use of fungicides to manage the pathogen generates contamination in the crops and residues in the harvested fruits. It is worth clarifying that it is prohibited to apply any product on the fruits during post-harvest because of the potential risk for consumers (EPPO, 2022; Palmieri et al., 2022).
There are reports of new Monilia strains that are tolerant and/or resistant to the active ingredients of some of the active fungicides, making their control increasingly difficult (FRAC, 2023). For example, there is resistance of an isolate of M. fructicola to the fungicide azoxystrobin (Chen et al., 2015). To mitigate the negative impacts of conventional agriculture, new biological control agents such as bacteria, fungi, and yeasts have been incorporated into the managment of diseases in different crops (Rudrappa et al., 2008). This represents a decrease in the use of chemical fungicides and a reduction in contamination and damage to the environment (Lima et al., 2011: Abbey et al., 2018).
Some bacteria, fungi, and yeasts naturally colonize the surface of plants, on their stems, leaves, fruits, and flowers. These organisms exert control over pest insects and phytopathogens, including Monilinia spp. (Palmieri et al., 2022). Bacillus is a cosmopolitan bacterium distributed in all agroecosystems. Bacillus arsenicus bacteria have been used in the bioremediation of soils and aquifers for arsenic (Shivaji et al., 2005), and as a biocontrol agent for fungi, phytopathogenic bacteria, and insect pests (biopesticides) (Elshakh et al., 2016). Numerous studies have shown that Bacillus species such as strains of Bacillus subtilis promote defense mechanisms in plants (Cesa-Luna et al., 2020). Other species of Bacillus affect different pathogens; their specificity depends on the production of antibiotics, biofilms, and siderophores that limit the growth of rhizosphere and phyllosphere microbiomes (Mosquera et al., 2014). Studies demonstrate the positive effect of B. subtilis in controlling tobacco root pathogens without affecting the soil microbiota (You et al., 2016).
Research shows the effectiveness of B. subtilis for the biocontrol of M. fructicola, both in field conditions and postharvest (Wang et al., 2018b; Palmieri et al., 2022). Part of the mechanisms used for its control is the production of lipopeptides, for example, phengimycin that has an antifungal effect (Yánez-Mendizábal et al., 2012). Other compounds developed by this bacterium are volatile organic compounds (VOCs) that inhibit the action of enzymes such as pectinase and cellulase, preventing cell damage caused by the pathogen (Zhou et al., 2019).
Some research shows the effectiveness of B. subtilis for the biocontrol of M. fructicola, both in field and postharvest conditions (Wang et al., 2018b; Palmieri et al., 2022). Among the mechanisms used for Bacillus subtilis CPA-8 to control M. fructicola is the production of lipopeptides, for example, phengimycin, which has an antifungal effect. Other compounds developed by this bacterium are volatile organic compounds (VOCs) that inhibit the action of enzymes such as pectinase and cellulase, preventing cell damage caused by peach brown rot (Monilinia spp.) (Yánez-Mendizábal et al., 2012).
Our research aimed to evaluate the control of M. fructicola in peach fruits under in vitro conditions and in fruits treated with two pre-selected isolates of Bacillus subtilis (CB10 and CB11) as biocontrol agents and the commercial fungicide a.i., dicloran.
MATERIALS AND METHODS
Microorganisms
In this research two Bacillus subtilis isolates were evaluated. These had previously demonstrated biocontrol activity against other phytopathogens. The CB10 isolate of B. subtilis Company. The CB11 and CB10 isolate of B. subtilis was isolated from a commercial peach crop located in Sotaquira (Boyaca) (Yuan et al., 2019). In the laboratory, CB11 was identified by morphological and biochemical tests (MacFaddin, 2003; Botero Ospina et al., 2013).
The Monilia fructicola isolate JEDO-107 used for this study was selected for its high pathogenicity, greater than >80% in four peach varieties. For the preparation of the biocontrol agent, each isolate of bacteria was seeded onto a glucose agar nutrient culture medium (5.0 g of peptone, 3 g of meat extract, 2.5 g of glucose, and 20 g of agar-agar in 1 L of distilled H20) and incubated for 3 d at 28°C. The isolate JEDO-107 of M. fructicola was cultivated onto a potato dextrose agar (PDA) medium for 15 d at 25°C.
Antifungal activity in vitro tests
To determine the in vitro antifungal activity of B. subtilis against M. fructicola, a dual culture method was used following Yuan et al. (2019). A M. fructicola mycelium disc (5 x 5 mm) was placed in the center of a PDA (pH = 7.4) Petri dish. To prepare the inoculum of the bacteria, the B. subtilis strains (CB11 and CB10) were cultivated in a liquid culture nutrient broth (pluripeptone 5 g L-1, meat extract 3 g L-1, sterile distilled water 1 L) for 3 d, accompanied by shaking and a temperature of 28°C. After incubation, CFU was counted in a Neubauer chamber; and the inoculum was prepared by adjusting it to a concentration of 1·109 CFU/mL, which is used for in vitro tests and for inoculation in peach fruits (Li et al., 2015).
For dual cultures, a loop of each isolate of B. subtilis was linearly streaked onto a PDA dish. A disk of mycelium of the fungus was then deposited in the center of the Petri dish. The inoculation of the bacteria and the fungus was carried out at the same time, and the dishes were incubated for 10 d at 25°C in total darkness.
Antagonist activity by bacteria was evaluated by the growth radius of the mycelium of M. fructicola vs. colonies of B. subtilis (CB10, CB11). The zone of inhibition around the mycelial disk of the pathogen was measured with a Vernier (Yuan et al., 2019). Measurements were carried out every 24 h for 8 d. The growth of the pathogen was compared to that of the control, where the bacteria were not present and the individual growth of the bacteria (Tab. 1).
TABLE 1. Treatments evaluated in vitro tests of B. subtilis against M. fructicola.
Treatments | Description |
---|---|
T1 C1 | Inoculation of isolate CB10, B. subtilis with M. fructicola onto PDA |
T1 C2 | Inoculation of isolate CB11, B. subtilis with M. fructicola, onto PDA |
C1 | Isolate CB10 of B. subtilis cultivated onto PDA |
C2 | Isolate CB11 of B. subtilis cultivated onto PDA |
T1 | Strain (JEDO-107) of M. fructicola cultivated onto PDA |
Antifungal activity in peach fruits
Healthy peach fruits var. Dorado were previously disinfected on the surface with a soapy water solution, and they were then immersed in a 2.5% sodium hypochlorite (NaClO) solution for 5 min. Finally, they were washed three times with sterile distilled water and dried under aseptic conditions in a laminar flow chamber.
Disinfected peach fruits were immersed in solution of each B. subtilis isolate (CB10, CB11) at a concentration of 1·10-9 CFU/mL, together with an adjuvant dispersant and adherent product [Inex-A, Cosmoagro, 0.3 g L-1] (active ingredient ethoxylated alkyl polyether alcohol, alkyl polyglycol, and aryl polyethoxyethanol), for 4 h (Li et al., 2015). Afterward, the fruits were wounded in the equatorial zone with a sterile loop; and a mycelium-agar disc (5×5 mm) of M. fructicola JEDO-107 was inoculated onto these fruits. The fruits were incubated for 15 d at 25°C in a humid chamber. Control fruits were inoculated with agar disks without fungus. The treatment was fruit inoculated with the pathogen. A chemical treatment with fungicide a.i. dicloran (BOTRAN 75 WP, Magro S.A) was included, where the same inoculation method described above was used. The mode of action of the fungicide was to inhibit the germination of conidia. The recommended dose for management of pathogens such as Monilia, Botrytis among others was 1.2 kg ha-1, according to efficacy tests (Tab. 2).
TABLE 2. Treatments evaluated in peach fruit for the control of M. fructicola.
Acronym | Description |
---|---|
TB1M | Inoculation with B. subtilis CB10 and M. fructicola |
TB2M | Inoculation with B. subtilis CB11 and M. fructicola |
TQM | Application of dicloran and inoculation of M. fructicola |
TM | Inoculation of M. fructicola |
TC | Inoculation s with sterile distilled water |
Treatment with Bacillus isolates was carried out as follows; first, the fruits were inoculated with the B. subtilis isolates (CB10 and CB11). Then with a sterile punch of 0.5 cm in diameter, perforations were made in the equatorial zone, and a disk of M. fructicola mycelium of the same size was deposited in each hole. Finally, in all the treatments evaluated, the fruits were left in humid chambers under controlled conditions at a temperature of 24°C and in total darkness for a period of 7 d. The experimental design was completely randomized with three repetitions. During the experiment, the percentage of severity was recorded, corresponding to the area of tissue affected by the disease and established according to the following formula and severity scale proposed by Zapata (2002) (Tab. 2).
The effect of control by isolates of B. subtilis and chemical fungicide was evaluated as the incidence and severity of brown rot on fruit. The growth of the area of lesion around the inoculation site was measured every 24 h for 8 d. The percentage of severity was also calculated according to a scale proposed by Zapata (2002). The inhibition rate was calculated according to formula Yuan et al. (2019) that evaluates the effectiveness of disease control (Eq. 1).
where, TI was inhibition rate (%), To lesion size of untreated fruits (cm2), Tt lesion size of treated fruits (cm2), and Tc lesion size of control (cm2) × 100%.
Statistical analysis
The experimental design was completely randomized with three repetitions for each treatment evaluated. In the first phase, the percentages of inhibition of the growth of the pathogen in the culture medium were calculated. In the second phase, the percentage of inhibition of the pathogen in fruits and the severity of the symptoms were calculated. Data were analyzed by applying ANOVA to determine the statistical differences between treatments with the LSD statistical test, using the SPSS Statistics 11.5 statistical package with a P<0.05.
RESULTS
Figure 1 shows peach fruits (Prunus persica [L.] Batsch), var. Dorado affected by brown rot caused by Monilia fructicola in the municipality of Jenesano (Boyaca-Colombia). Infected plants show circular spots; then a dense layer of gray mycelium forms that quickly covers them causing rot and finally mummification (loss of water from the tissues). The affected fruits lose all commercial value and are a source of pathogen inoculum. The isolation of M. fructicola (JEDO-107), was via the culture medium potato dextrose agar (PDA). The mycelium shows abundant sporulation with gray and yellow in the center and was identified by molecular and pathogenic tests causing severity > 80% in four peach varieties (Guarín Torres et al., 2019).
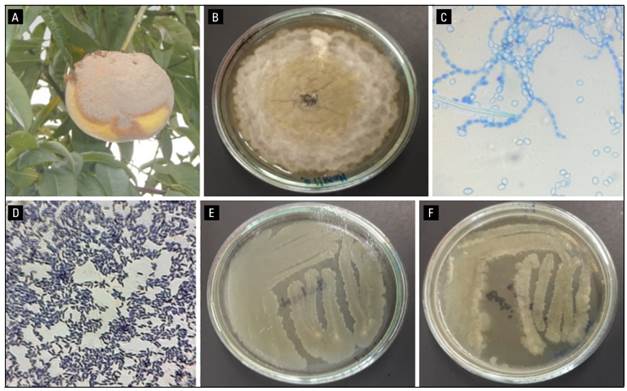
FIGURE 1. Characteristics of pathogen fungus and biocontroller bacteria. A, peach fruit infected with brown rot was used for this study. B, colony of Monilinia fructicola JEDO107 onto PDA medium grown at 25°C and 96 h. C, morphology of ramoconidia of Monilia seen under the microscope at 40x. D, Bacillus subtilis after Gram staining viewed at 100x. E, Bacillus subtilis isolate CB10 on nutrient agar after 48 h. F, Bacillus subtilis isolate CB11 onto nutrient agar after 48 h.
Two isolates of Bacillus spp. (CB10 and CB11) were identified in the laboratory. The colonies were white in color, mucoid in appearance, and measured approximately 2.5 to 4.0 mm in diameter. Their appearance was smooth; the edges were wavy; their growth was very rapid in nutrient agar (NA) culture medium. After 24 h, gram staining was performed and they were identified as gram-positive bacilli; their shape was bacillary, each measuring 1 to 6 µm long, with spores in the center of the bacteria (Fig. 1).
Antifungal activity in vitro tests
We evaluated the biocontrol capacity of B. subtilis CB10 and CB11 against M. fructicola by the inhibition of mycelial growth and its halo. Monilia fructicola had a mycelial inhibition rate close to 90%, and there was also evidence of a reduction in the sporulation. The CB11 isolate inhibited 65% of the fungus pathogen and the inhibition halo was less than 7.5 mm.
According to the results, a decrease in the diameter of the mycelium and sporulation of the pathogen was recorded in fruits inoculated with the two isolates of B. subtilis CB10 and CB11 accompanied by a commercial fungicide dicloran compared to the control (Fig. 2). There were differences in the size of the inhibition zone between the confrontation of each B. subtilis isolate and the growth of the fungus on an agar surface. This is shown in the biocontrol effect of B. subtilis CB11 against this phytopathogen compared to B. subtilis CB10 (Tab. 3).
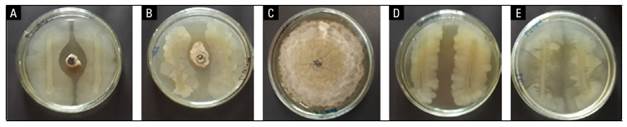
FIGURE 2. In vitro test of Bacillus subtilis for the control of Monilinia fructicola JEDO-107 a) strain CB10 of B. subtilis x M. fructicola; b) strain CB11 of B. subtilis x M. fructicola; c) M. fructicola (JEDO-107); d) B. subtilis strain CB10; e) B. subtilis strain CB11. In vitro test at 25°C for 96 h.
TABLE 3. Rate and halo of inhibition of two strains of B. subtilis against M. fructicola.
Treatments | Inhibition rate (%) | Inhibition halo size (mm) |
---|---|---|
M. fructicola x B. subtilis CB10 | 88.5±1.73 | 14±1.41 b |
M. fructicola x B. subtilis CB11 | 70±1.41 | 7.5±1.29 a |
The meaning of different letters showss statistical differences according to the LSD test, P<0.05.
Antifungal activity in peach fruit
The evaluated concentration of 1·109 CFU/mL allowed control of M. fructicola. It should be noted that the of B. subtilis CB10 showed better control than B. subtilis CB11 while CB11 registered similar results of control to fungicide. Results show that applications of the B. subtilis CB10 can be used to control brown rot caused by M. fructicola (Fig. 3).
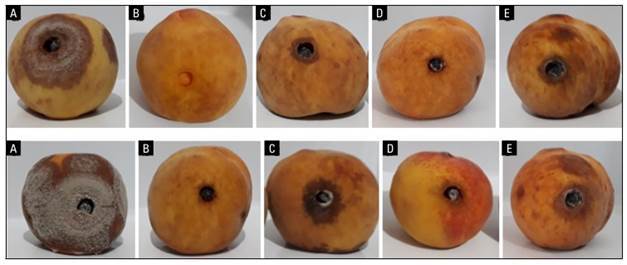
FIGURE 3. Antifungal effect of isolates of B. subtilis (CB10 and CB11) and a fungicide dicloran against the isolate JEDO-107 of M. fructicola in peach. fruit. Fruit symptoms at 3 d (above) and 6 d (below), where: a, fruit inoculated with M. fructicola; b, absolute control; c, CB11 isolate with M. fructicola; d, CB10 isolate with M. fructicola. e, fruits sprayed with dicloran and with M. fructicola.
The results showed that when the fruits were inoculated with M. fructicola, the disease developed very quickly, affecting more than 60% of the surface of the fruits in less than 6 d. In the fruits treated with the fungicide dicloran, the disease affected up to 35% of the fruit surface, and a similar result was registered with B. subtilis CB11 compared to the control fruit. Finally, with the treatment with the B. subtilis CB10, the lowest severity percentage on fruit was recorded, with only 10% (Figs. 3 and 4).
Rot inhibition rate
To determine the control of M. fructicola exerted by two isolates of B. subtilis and the fungicide Dicloran, we evaluated the inhibition rate corresponding to the effect of growth on the phytopathogen. The results showed that the treatment corresponding to the CB10 isolate was the most effective in the control of the disease, registering a lower average growth radius of 0.53 mm per day and a higher rate of inhibition with high statistical differences > 95. The inhibition rates for the treatments corresponding to Dicloran and B. subtilis CB11 were similar without statistical differences. For the treatment with fruit inoculated with the pathogen, the average growth radius was 4.53 mm d-1. The sporulation demonstrates the fast multiplication of the pathogen in the fruits. This shows that there was an anti-sporulating and antifungal effect produced by the B. subtilis strains (Tab. 4).
TABLE 4 Inhibition rate of M. fructicola and mean radius of the lesion in peach fruits treated with two strains of B. subtilis (CB10 and CB11) and a fungicide.
Treatments | Rate of inhibition (%) | The mean radius of the lesion (mm) |
---|---|---|
M. fructicola | - | 21.16±4.53 a |
Dicloran | 70.33±14.43 a | 20.13±2.32 b |
B. subtilis (CB11) | 71.33±13.65 a | 4.61±2.46 b |
B. subtilis (CB10) | 95.83±7.21 b | 5.77±0.53 c |
Different letters show statistical differences according to the LSD test, P<0.05.
DISCUSSION
Biological disease control is a new approach to the management of the main diseases in crops of interest. In recent years, the effect of the applications of Bacillus strains on the phyllosphere and rhizosphere of plants has been studied (Rudrappa et al., 2008). and different members of the Bacillus genus are known to benefit plants by protecting plants from pathogens like Botritys cinerea and Cladosporium fulvum (Wang et al., 2018a). Different species of Bacillus called plant growth-promoting bacteria (PGP) are being used in the manufacture of new biofertilizers and as agents for use in sustainable agriculture (Wang et al., 2018b).
Bacillus subtilis is the first bacteria reported as a biological controller of brown rot (M. fructicola) (Wint) Honey, in peach fruits (Wilson and Wisniewski, 1989). Numerous antibiotics and lipopeptides have been identified such as: fengycin, iturin and surfactin that this bacterium produces to control this pathogen (Ongena et al., 2005). Work done by Yánez-Mendizábal et al. (2012) with the CPA-8 strain of Bacillus subtilis, demonstrated strong antifungal activity against two isolates of M. Laxa and M. fructicola.
One of the indirect mechanisms developed by different Bacillus species is to increase systemic resistance in plants (Elshakh et al., 2016), the solubilization and mineralization of nutrients such as phosphorus and potassium, nitrogen fixation (Rudrappa et al., 2008), the production of 1-aminocyclopropne-1-carboxylic acid (ACC), antagonists of pathogens (Yuan et al., 2019), phytohormones and antimicrobial compounds (Gotor-Vila et al., 2017), and the stimulation of plant defenses (Passari et al., 2018; Etesami et al., 2023). Another of the control mechanisms against reported pathogens are direct competition for space and nutrients, parasitism, antibiosis, and the production of enzymes and siderophores (Wong et al., 2016; Zhu et al., 2023; Reyna et al., 2023). Bacillus species antagonize various plant diseases induced by nematodes, fungi, viruses, bacteria, and other plant pests (Etesami et al., 2023).
In other research, antagonistic effects of different isolates of Bacillus spp. against certain phytopathogens such as M. fructicola were explained by the production of fengycin-like lipopeptides such as sulfactins, bacilomycins D, iturin A, and fengimycin with antifungal activity (Yánez-Mendizábal et al., 2012). The fengimycin produced by the strain CPA-8 of Bacillus subtilis controlled by M. fructicola (Yánez-Mendizábal et al., 2012). In other research using the strain WXCDD105 of B. subtilis, compounds such as antibiotics and chitinases reduced the severity caused by Botrytis cinerea and Cladosporium fulvum in tomato fruits (Wang et al., 2018a). Antibiotics, produced by B. subtilis, play a very important role in the control of phytopathogens. There is direct evidence from in vitro tests that they inhibit or slow down growth as an important biocontrol mechanism (Patiño et al., 2012; Mosquera et al., 2014). Another compound that stands out in the biocontrol of fungi is volatile organic compounds (VOCs). Zhou et al. (2019) describe the use of the CF-3 strain of B. subtilis against M. fructicola in vitro and in vivo tests.
In studies developed by Zhen el al. (2022), we demonstrated that the CF-3 strain of B. subtilis produced compounds such as VOC CF-3 that influenced reducing the pathogenicity and growth of M. fructicola, in affected fruits. This is the basis for a potential mechanism of action against this pathogen.
CONCLUSIONS
We demonstrated that the CB10 strain of B. subtilis can be used as a preventive control for M. fructicola. This strain inhibited the growth and sporulation of the pathogen both in vitro tests and in inoculated fruits. This research demonstrated the potential of the B. subtilis CB10 strain as a biological control against M. fructicola. It is important to evaluate the interaction of B. subtilis with other bacterial strains, to use them as biofungicides or bioinoculants in different crops and deciduous trees.